Research on radiation level measurement in mobile accelerator sites
Highlight box
Key findings
• Our results indicated higher dose levels at distances from the center compared to previous studies, suggesting a lower proportion of leakage radiation. The primary contributor was found to be the induced radiation from scattered electrons in the scattering body.
What is known and what is new?
• Studies by Hua et al. and Ma et al. using thermoluminescent dosimeter (TLD) measurements around intraoperative radiotherapy (IORT) accelerators reported dose rates consistent with this study’s findings.
• Our study, measuring dose levels in different directions and distances on the treatment bed plane, provided reference values for IORT shielding design and personnel protection. Key features of our study include adjusting detection points’ distances to minimize scattered radiation effects and estimating dose rates above the treatment bed plane.
What is the implication, and what should change now?
• Limitations include the inability to measure axis 5 due to obstacles and the absence of detection points below the treatment bed plane to account for backscatter. Despite these limitations, our TLD measurement system, calibrated with irradiator and fixed linear accelerator electron beams, offers valuable insights into the radiation field during mobile accelerator radiotherapy.
• Future studies can expand the scope to include various radiotherapy equipment for comprehensive shielding design references.
Introduction
Intraoperative radiotherapy (IORT) has a history of over a century in treating tumors. Traditional IORT, employing medical electron linear accelerators used for routine radiotherapy, poses risks such as infection and anesthesia accidents to patients during the treatment process. The introduction of mobile electron linear accelerators has made it feasible to conduct IORT in the operating room, addressing some concerns but posing challenges for radiation protection in IORT operating rooms.
In this study, we focused on a Mobetron mobile accelerator, conducting measurements and analysis of the radiation field’s dose levels and on-site protection during the accelerator’s operational state using thermoluminescent dosimeters (TLDs). The aim is to provide insights into the radiation shielding design of mobile accelerators in the context of IORT.
Previously, studies by Hua et al. (1) and Ma et al. (2) have investigated the dose rates around mobile accelerators. In this study, the research location and auxiliary medical equipment involved have all been fully equipped, resulting in dose measurements that are closer to real-life conditions. Additionally, quality control measures were implemented for the research material—TLDs, ensuring the accuracy of the data.
Methods
Study object
The research focuses on a mobile accelerator, model Mobetron, installed in an operating room of a medical institution. The accelerator utilizes electron beams for IORT, with three energy levels: 6, 9, and 12 MeV. The accelerator head can rotate along the longitudinal plane by 30° using a C-arm. The source-to-skin distance is 50 cm. A collimator is placed below the head to shield radiation passing through the irradiated body.
Thermoluminescent dosimetry system
Harshaw 5500 Thermoluminescent Dosimeter (Thermo Electron Corporation, USA), LAB-01 Annealing Furnace (Industrieofenbau and Thermotechnik GmbH, Germany), and LiF (Mg, Cu, P) Thermoluminescent Detectors (Beijing Kankeluo Corporation, China). All instruments undergo calibration in the secondary standard dosimetry laboratory of the China Institute of Metrology Science before use.
Detection conditions
The experiment employs the Mobetron mobile accelerator with 12 MeV electron beams.
Irradiation conditions: dose rate of 300 cGy·min−1, accelerator head set in a vertical position, a 10 cm diameter collimator installed. In order to simulate the interaction between patient scatter during treatment and the surrounding equipment rack materials, we placed a water phantom measuring 30 cm × 30 cm with a depth of 30 cm below the collimator.
Total irradiation dose: 60 Gy over a total irradiation time of 20 min.
Detection point configuration
Using the beam axis as the z-axis, on the treatment bed plane, points are set around the beam axis in a radial pattern every 30°. To minimize the impact of scattered radiation from various items in the operating room, detection points are set at distances of 100, 110, 125, 145, 200, and 300 cm along each axis (equidistant from the center). Results from each detection point are used to calculate the dose level at 1 m from the center using the inverse square distance formula, with the mean of these results considered as the final detection result.
The distribution of detection points on the treatment bed plane is shown in the figure, and the schematic diagram of detection points is illustrated in Figure 1.
The interaction between the electron beam and the water phantom generates X-rays within a cylindrical volume. The height of this cylinder is similar to the range of 12 MeV electrons in the water phantom, approximately 6 cm. The cross-section of the cylinder matches the dimensions of the collimator, forming a circle with a diameter of 10 cm. The distances between the detection points set in this study and the geometric center of this cylinder are significantly greater than five times the geometric dimensions of any individual radiation source, satisfying the condition of considering the radiation source as a point source.
To observe the radiation levels above the accelerator, four axial lines are drawn at a 45-degree angle above the treatment bed plane along axes 3, 6, 9, and 12, starting from the central point. Along each axial line, detection points are placed at radial distances of 141 cm (projected onto the treatment bed plane at a distance of 100 cm from the center), 155 cm (projected onto the treatment bed plane at a distance of 110 cm from the center), 176 cm (projected onto the treatment bed plane at a distance of 125 cm from the center), and 204 cm (projected onto the treatment bed plane at a distance of 145 cm from the center). The radiation levels at each detection point are calculated using the inverse square law formula to determine the radiation level at a distance of 1 m along the radial direction from the center, and the average of the calculated results from all detection points is considered as the final measurement result.
Due to the interaction between the electron beams and the water phantom, the range extends to a cylindrical shape with a diameter of approximately 10 cm and a height of about 6 cm, and the geometric center of Figure 1 is offset from the actual geometric center of the range of X-rays generated during irradiation, the calculation of the dose rate at 1 m on each axis involves some adjustments. The 6th and 12th axes are set as the x-axis, while the 3rd and 9th axes are set as the y-axis. When applying the inverse square law to calculate the dose rate at 1 m, adjustments were made for the actual spatial position of the central point.
Quality control
Considering that the vicinity of the mobile accelerator is a mixed field containing both leakage radiation and induced radiation, encompassing both photons and electron beams, it is essential to ensure that the readings obtained from TLDs accurately reflect the dose levels at the detection points.
To achieve this, the linearity of TLD responses to both photons and electron beams needs to be understood. The method involves irradiating TLDs at different doses using an irradiator and a medical electron linear accelerator. Detection readings are recorded, and dose-response curves are plotted, with the incident dose as the x-axis. Linear analysis is then performed.
For the irradiator, irradiation conditions are as follows:
- Activity: 10 Ci.
- 137Cs source.
- TLD positioned 54.7 cm from the radiation source.
- Irradiation doses: 0.3, 1, 5, 10, 20, and 30 mGy.
For the linear accelerator, irradiation conditions are as follows:
- Electron beam energy: 9 MeV with a source-to-skin distance of 100 cm.
- TLDs were placed at the maximum dose depth underwater.
- Irradiation doses: 10, 20, 50, 100, 200, and 300 mGy.
Data analysis
The processing of dose results at specific locations
To ensure the accuracy of dose results at each location, we deployed i TLD elements at each location. After irradiation, the reading Mji of the j-th TLD element at the specific location is obtained from the reader. Then, the average reading at that location can be calculated
represents the reading of the -th TLD element at the specific location.
represents the average reading at that location.
The standard deviation of the readings at that location can be calculated as follows:
Substituting the average and standard deviation of the -th location into the linear relationship equation of the electron beam to obtain the final dose result and dose deviation.
Radiation source position correction
In this study, the primary radiation source is X-rays generated by the interaction of the electron beam with the phantom. The X-ray range has a diameter of 10 cm (diameter of the collimator), and its height forms a cylinder corresponding to the range of the electron beam in water. Due to the geometric dimensions of the radiation source not being strictly point sources, there is an offset between the detection point and the geometric center of the actual source (with an offset of in the X direction and in the Y direction). Therefore, when calculating the dose rate at 1 m on each axis, position correction is required.
Setting the 6th and 12th axes in Figure 1 as the x-axis, and the 3rd and 9th axes as the y-axis, the dose results at different distance points () on each axis are denoted as . The radiation source position can be corrected using the following equation
where is a constant value.
Results
TLD detector responses to photons and electron beams
TLDs were irradiated with various doses using an irradiator, with doses of 0.3, 1, 5, 10, 20, and 30 mGy. To ensure consistency in radiation quality, a medical electron linear accelerator with a 9 MeV electron beam was also used to irradiate TLDs with doses of 10, 20, 50, 100, 200, and 300 mGy. X-ray and electron beam readings were recorded, and dose-response curves were plotted with incident dose as the x-axis. Linear analysis was performed. The linear relationship between the reading after accelerator irradiation and the reading after irradiation with the irradiation dose is as follows:
and both curves showed a correlation coefficient of 0.999.
Intraoperative radiation levels in the radiotherapy operating room
The readings of the TLD elements after irradiation from both the accelerator and the irradiator in Figure 2 show good linearity with the dose. Additionally, the slopes of the dose curves for both are similar. In Eq. [4] of this study, the slope 1,244 serves as the conversion factor between the readings of the TLD elements and the dose. Further, the dose results at each point are converted into dose rates using an irradiation time of 20 minutes. TLDs were utilized to detect dose rates at various points on the treatment bed plane, 100 cm from the center, as shown in Table 1. Axis 5 was not measurable due to obstruction by the accelerator body and proximity to the room wall. Results indicated that, except for axis 6, dose rate estimates from various detection points, 100 cm from the center, ranged from 39 to 50 mGy/h. Axis 6 exhibited lower results mainly due to obstruction by the accelerator body.
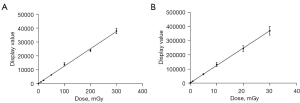
Table 1
Number of axis | Angle with the axis of the accelerator (°) | Dose rates at different distances from the center point (mGy/h), mean ± SD | Dose rate calculation result at 100 cm (mGy/h), mean ± SD | |||||
---|---|---|---|---|---|---|---|---|
1 m | 1.1 m | 1.25 m | 1.45 m | 2 m | 3 m | |||
1 | 315 | 46.0±7.1 | 27.5±2.8 | 27.7±0.7 | 20.2±3.3 | 9.8±0.8 | 4.5±0.3 | 43.2±11.6 |
2 | 285 | 27.7±6.6 | 25.6±4.0 | 28.4±4.5 | 19.8±1.7 | 12.0±1.4 | 4.8±0.3 | 47.8±17.4 |
3 | 255 | 36.4±2.8 | 28.1±1.5 | 23.4±2.3 | 19.0±1.5 | 7.7±0.4 | 4.6±1.0 | 38.9±10.7 |
4 | 225 | 25.7±2.8 | 32.3±2.8 | 15.9±1.8 | 11.5±2.0 | 8.8±1.8 | –† | 43.6±14.1 |
5 | 195 | –† | –† | –† | –† | –† | –† | –† |
6 | 165 | 10.7±1.2 | 2.1±0.4 | 1.1±0.2 | 1.0±0.03 | 0.9±0.1 | –† | 5.4±1.6 |
7 | 135 | 44.7±1.2 | 30.2±2.6 | 22.4±0.7 | 14.2±1.8 | 9.4±0.9 | –† | 38.6±7.1 |
8 | 105 | 28.4±5.3 | 33.5±3.8 | 25.8±0.4 | 14.3±4.5 | 9.8±0.5 | –† | 41.0±15.8 |
9 | 75 | 48.7±4.6 | 39±5.9 | 17.6±3.1 | 21.5±1.9 | 8.0±1.0 | –† | 44.6±13.0 |
10 | 45 | 38.1±1.8 | 39.9±2.7 | 28.2±3.1 | 23.3±2.9 | 7.1±2.0 | 5.5±0.1 | 45.4±15.4 |
11 | 15 | 49.1±5.5 | 32.9±5.1 | 23.3±2.4 | –‡ | 6.6±0.6 | 3.5±0.2 | 39.2±9.5 |
12 | 345 | 56.3±5.5 | 45.2±3 | 26.4±3.3 | 24.8±2.6 | 8.4±0.5 | 3.4±0.5 | 47.3±12.0 |
†, indicates that measurement points could not be set due to limitations in the length and width of the room; ‡, indicates that the thermoluminescent element fell off at that location, resulting in no measurement data. SD, standard deviation.
Dose attenuation
Axis 6, situated at the rear of the equipment, showed significantly lower results compared to other detection axes. The average of the measurements at points with the same distance along each axis, excluding axis 6, is plotted against the distance from the center point on the x-axis, with the average measurement values of points at the same distance plotted on the y-axis. The results are depicted in Figure 3. Dose levels decreased with increasing distance from the center, reaching 6.9 mGy/h at 300 cm from the center.
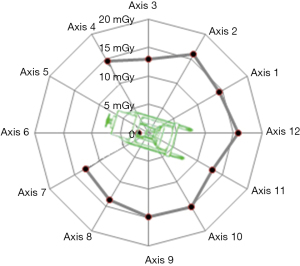
Comparison of dose levels at different heights
To observe the dose levels in the upper region of the mobile accelerator, axes were drawn at 45° angles above the treatment bed plane along axes 3, 6, 9, and 12. Dose levels at different distances from the center are presented in Table 2. Results indicated higher readings for axes 9 and 12 in front of the accelerator and lower readings for axes 6 and 3 behind the accelerator.
Table 2
Number of axis | Angle with the axis of the accelerator (°) | Dose rates at different distances from the center point (mGy/h), mean ± SD | Results of dose rate at a radial distance of 100 cm (mGy/h), mean ± SD | |||
---|---|---|---|---|---|---|
1.41 m | 1.55 m | 1.76 m | 2.05 m | |||
3 | 255 | 3.8±0.5 | 3.5±0.1 | 3.1±0.2 | 2.6±0.1 | 9.1±1.3 |
6 | 165 | 3.8±0.4 | 1.4±0.2 | 1.1±0.1 | 0.8±0.1 | 9.5±2.0 |
9 | 75 | 1.6±0.1 | 1.8±0.4 | 1.3±0.1 | 0.8±0.1 | 11.1±2.8 |
12 | 345 | 2.3±0.2 | 1.4±0.3 | 1.1±0.1 | 1.0±0.1 | 11.8±3.1 |
SD, standard deviation.
Discussion
IORT is relatively less utilized compared to conventional radiotherapy due to limitations in equipment and facility protection. Research on its protection measures is relatively scarce (1-6). In a study by Hua et al. (1), the dose rate around a specific IORT accelerator was measured using TLD. Their study irradiated for 1 minute at a dose rate of 10 Gy/min, resulting in a dose of approximately 0.7 mSv at 100 cm from the center point, corresponding to a dose rate of about 42 mSv/h, which is similar to the findings of this study. Related research by Ma et al. (2) also indicated dose rates ranging from 40.8 to 62.8 mSv/h in front of the IORT accelerator at 100 cm, while behind it, the dose rates ranged from only 0.13 to 11.2 mSv/h, which is comparable to the results of this study.
Research on mobile accelerators has also been conducted abroad, with studies by Daves et al. (7) and Krechetov et al. (8) focusing on measuring the leakage radiation from the Mobetron mobile accelerator. The study by Krechetov et al. (8) revealed that on a plane of Z =100 cm, the leakage radiation level at 300 cm exhibited a trend of being higher in the front than in the rear, ranging from 2.2 to 4.5 µGy/10 Gy. Based on the results of this study, the radiation level at a distance of 300 cm from the center point of the treatment bed plane could reach 100–885 µGy/10 Gy, significantly higher than the leakage radiation levels reported in the aforementioned study. This indicates that in the radiation field surrounding the mobile accelerator, the proportion of leakage radiation and scattered radiation is relatively low, with the main contribution likely coming from the secondary radiation generated by the therapeutic electron beam in the scatterer.
In addition to measuring the dose distribution within the treatment bed plane, this study also measured partial dose levels along axes inclined at a 45° angle from the center point of the treatment bed plane, aiming to provide partial reference for shielding above the treatment bed during IORT. Measurements along four axes inclined at a 45° angle from the horizontal plane of the treatment bed resulted in calculated dose rates ranging from 9.1 to 11.8 mSv/h at a radial distance of 100 cm from the center point. These values are significantly lower than the dose rates observed on the treatment bed plane. Similar results were reported by Hua et al. (1), indicating that the maximum dose occurs on the treatment bed plane, while dose rates at points above the treatment bed plane are noticeably lower than those on the treatment bed plane.
This study measured the dose levels at different distances and directions from the treatment bed plane and calculated the dose rates at 100 cm from the center point in each direction, providing a reference basis for shielding and arranging protection for personnel during IORT. The study has the following characteristics: (I) due to the completion of the radiotherapy operating room renovation and the installation of various surgical equipment and instruments, the scattered radiation from these objects inevitably affects the measurement results. Therefore, the study placed detection points at different distances along each axis to collectively estimate the dose rate at 100 cm from the center point in each direction, aiming to minimize the impact of scattered radiation on the estimated results; (II) the study measured some detection points on planes inclined at a 45° angle from the treatment bed plane and roughly estimated the dose rate above the treatment bed plane; (III) the TLD detectors used in this study were calibrated using irradiators and fixed linear accelerator electron beams. Linear analysis results showed good linearity, with slopes close to unity, indicating that the TLD detectors used in this study exhibited good responsiveness to both electron beams and X-rays.
This study also has limitations, primarily including: (I) due to obstruction by the accelerator frame and proximity to the wall, detection points could not be placed behind axis 5, located at the rear side of the accelerator, resulting in no measurement results obtained; (II) considering backscatter from the shielding floor, detection points were not set below the treatment bed plane.
Conclusions
This study utilized a TLD measurement system verified by irradiators and accelerator electron beams to measure and estimate the radiation field dose levels during mobile accelerator radiotherapy, providing reference data for IORT radiation protection shielding. In the future, expanding the scope of research to measure and estimate the surrounding dose distribution during treatment with other radiation therapy devices could provide further basis for shielding designs of more radiotherapy equipment.
Acknowledgments
Funding: This study was supported by
Footnote
Data Sharing Statement: Available at https://jphe.amegroups.com/article/view/10.21037/jphe-24-7/dss
Peer Review File: Available at https://jphe.amegroups.com/article/view/10.21037/jphe-24-7/prf
Conflicts of Interest: All authors have completed the ICMJE uniform disclosure form (available at https://jphe.amegroups.com/article/view/10.21037/jphe-24-7/coif). The authors have no conflicts of interest to declare.
Ethical Statement: The authors are accountable for all aspects of the work in ensuring that questions related to the accuracy or integrity of any part of the work are appropriately investigated and resolved. No patients were involved in this study. Neither approval from the Ethics Committee nor informed consent from participants was required.
Open Access Statement: This is an Open Access article distributed in accordance with the Creative Commons Attribution-NonCommercial-NoDerivs 4.0 International License (CC BY-NC-ND 4.0), which permits the non-commercial replication and distribution of the article with the strict proviso that no changes or edits are made and the original work is properly cited (including links to both the formal publication through the relevant DOI and the license). See: https://creativecommons.org/licenses/by-nc-nd/4.0/.
References
- Hua H, Liu H, Wu J, et al. Experimental research of dose distribution and protection for mobile intra-operative radiotherapy accelerator in operating room. Chia J Raodiol Med Prot 2012;32:652-5.
- Ma Y, Wan L, Lou Y, et al. Measurement and analysis on the radiation level of Intra-operative radiotherapy(IORT) with a mobile accelarator. Capital Journal of Public Health 2012;6:150-4.
- Ciocca M, Pedroli G, Orecchia R, et al. Radiation survey around a Liac mobile electron linear accelerator for intraoperative radiation therapy. J Appl Clin Med Phys 2009;10:131-8. [Crossref] [PubMed]
- Chen Y, Zhang D, Liao X, et al. Measurement of the neutron dose equivalent rate from a dedicated intraoperative radiation therapy accelerator. Chia J Raodiol Med Prot 2018;38:307-10.
- Han C, Yan Y, Chen C, et al. Analysis of radiation levels in a workplace using an intraoperative radiotherapy accelerator. Chin J Radiol Health 2012;73-5.
- Ju Z, Gong H, Ren S. Leakage Doses of a Mobile Accelerator Around an Operation Room (Mobetron). Chinese Medical Equipment Journal 2011;32:107-9.
- Daves JL, Mills MD. Shielding assessment of a mobile electron accelerator for intraoperative radiotherapy. J Appl Clin Med Phys 2001;2:165-73. [Crossref] [PubMed]
- Krechetov AS, Goer D, Dikeman K, et al. Shielding assessment of a mobile electron accelerator for intra-operative radiotherapy. J Appl Clin Med Phys 2010;11:3151. [Crossref] [PubMed]
Cite this article as: Xu X, Du X, Yang C, Wang J. Research on radiation level measurement in mobile accelerator sites. J Public Health Emerg 2024;8:21.