Outcomes and development of new cardiovascular conditions in patients with pre-existing cardiovascular disease after contracting COVID-19
Highlight box
Key findings
• Having cardiac comorbidities results in worse disease outcomes including higher mortality rates, requiring higher levels of care, and longer hospital stays. Having comorbidities and receiving higher levels of care also increases the likelihood of developing a new cardiovascular disease (CVD).
What is known and what is new?
• Many studies have reported a significant association between pre-existing CVD and severe outcomes in coronavirus disease 2019 (COVID-19) patients.
• This study considers a wide variety of CVDs and their effects on the outcome of COVID-19 patients, as well as how patients fare in terms of developing new conditions post-infection.
What is the implication, and what should change now?
• This corroborates the understanding that COVID-19 can have adverse outcomes in those with CVD, therefore, these patients should receive specially tailored care.
Introduction
The COVID-19 pandemic has presented an array of unprecedented challenges for the general population. The global impact of the pandemic has created an urgent need for comprehensive research regarding its multifaceted public health consequences. As of October 2023, COVID-19 has 767,750,853 confirmed cases worldwide, with 6,941,095 deaths (1). At its best, individuals who have contracted the virus recover swiftly; however, COVID-19 may result in serious complications.
One risk factor for adverse disease outcomes is having comorbidities. Among those affected by COVID-19, 94.9% of hospitalized patients and 47% of patients who have died had at least one COVID-19 related comorbidity with the most common ones being hypertension, cardiovascular disease (CVD), diabetes, and obesity (2-4). The increasing number of COVID-19-related deaths, especially among patients with comorbidities, has brought attention to the impact of COVID-19 on the cardiovascular system. Although COVID-19 manifests in many organ systems, it has been shown to be particularly taxing on the cardiovascular system, thereby exacerbating any pre-existing CVD conditions and eliciting complications that result in a more severe disease progression and an increased risk of mortality (5).
With CVDs being a leading cause of global morbidity and mortality, it is vital to expand our knowledge on how COVID-19 infection may exacerbate outcomes. Numerous studies have reported a significant association between pre-existing CVD and adverse outcomes in COVID-19 patients. A meta-analysis found that the pooled prevalence of preexisting CVD and hypertension in hospitalized COVID-19 patients was 18.2% and 32.7%, respectively (6). Many other studies investigating the impact of specific cardiovascular comorbidities on COVID-19 outcomes have provided valuable insights into specific types of CVD. Hypertension has been identified as the most common comorbidity by numerous studies and was associated with higher mortality rates (7). Similarly, heart failure, atrial fibrillation, venous thromboembolism, peripheral vascular disease, cerebrovascular disease, coronary artery disease, and a history of myocardial infarction (MI), are also among many significant predictors of a tougher disease course and increased mortality (8-12).
The observed trend of COVID-19 exacerbating disease severity in patients with pre-existing cardiovascular conditions and inducing new CVD is important to consider in the context of the many effects that COVID-19 has. This study aims to investigate the correlation between cardiovascular comorbidities and patient outcomes in terms of the 30-day mortality rate, highest level of care required [self-managed care, hospital admission, or intensive care unit (ICU) admission], length of hospital stay, and development of new cardiovascular diagnoses within the rural population of The Guthrie Clinic (TGC). Understanding the outcomes faced by this vulnerable population is crucial for developing well-suited prevention and management strategies and for optimizing their care and health outcomes. We present this article in accordance with the STROBE reporting checklist (available at https://jphe.amegroups.com/article/view/10.21037/jphe-24-22/rc).
Methods
This was a retrospective cohort study conducted within TGC, a health system located in north central Pennsylvania and upstate New York. TGC has hospitals in Sayre, PA, Corning, NY, Towanda, PA, Troy, PA, and Cortland, NY, and sees upwards of 1,500,000 patient visits each year, most of them coming from rural communities.
This study consisted of patients 18 years or older who tested positive for COVID-19, confirmed by a polymerase chain reaction (PCR) test during the 2020 calendar year—from March 2020 (when the first positive COVID-19 test was recorded within the health system) until December 31, 2020. This included those who underwent self-managed recovery without requiring hospital admission and those who required inpatient hospital admission and had a positive test within 2 weeks of their admission date (14 days before or after). The cut-off was set at 14 days to accommodate both the duration of symptomatic illness and the incubation period of COVID-19. The cutoff threshold was also extended up to 14 days after admission since PCR results may initially be negative during the early incubation period of the virus (13). Patients were excluded if their hospital or ICU admission date was after December 31, 2020, even if their initial positive COVID-19 test was prior.
Data were collected via the Epic electronic medical record. Data points included demographic information, dates of positive COVID-19 test, hospital admission, hospital discharge, ICU admission, ICU discharge, mortality status, and cardiac comorbidities. Demographic information included age, gender, and ethnicity. Cardiac comorbidities were defined in terms of whether the patient had them before their initial COVID-19 diagnosis or if they developed after COVID-19 infection. Cardiac comorbidities were identified with ICD-10 (International Classification of Diseases, 10th revision) codes (Table 1). These comorbidities were chosen because they are common cardiovascular comorbidities and were frequently considered in similar studies (14,15). To assess the more long-term implications of COVID-19 infection, we also gathered data on the development of new cardiac conditions, as defined by the same ICD-10 codes, up until December 2023.
Table 1
Condition | ICD-10 code |
---|---|
Hypertension | I10 |
Arrhythmia | |
Atrial fibrillation and flutter | I48 |
Ventricular arrhythmia | I49 |
Myocardial infarction | I21−I22 |
Stroke | I60−I69 |
Pericarditis | I30, I31, I32 |
Myocarditis | I40, I41, I51.4 |
Ischemic heart disease | |
Acute coronary disease | I24 |
Myocardial infarction | I21, I22 |
Ischemic cardiomyopathy | I25.5 |
Angina | I20 |
Heart failure | I50 |
Thrombotic disorders | |
Pulmonary embolism | I26 |
Deep vein thrombosis | I80.1, 80.2, I81, I82.0, 82.2, 82.3, 82.4, 82.5 |
ICD-10, International Classification of Diseases, 10th revision.
Due to the established association between tobacco smoking and CVDs, we decided to gather data on smoking habits at the time of their first positive COVID-19 test. We categorized patients as a “non-smoker”, “former smoker”, or “current smoker”. We defined a “non-smoker” as an individual with no smoking indicated in their patient history. We defined a former smoker as one whose patient history indicated that they stopped smoking before their initial COVID-19 diagnosis. A current smoker was defined as someone who had not quit smoking at the time of their COVID-19 diagnosis.
The working sample size was 4,688 patients after the application of the inclusion and exclusion criteria. A flowchart of how the final cohort was determined is depicted in Figure 1. Patients were excluded if they were under 18 years old or were reinfected with COVID-19 during the study period. Reinfection was defined as having a positive test over 90 days after the first positive test (16).
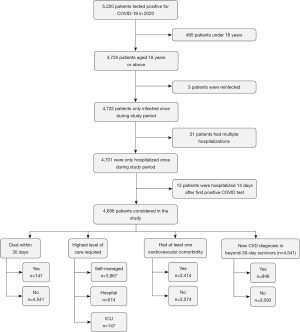
There were also two specific sets of patients that were excluded from this study. Twenty-one patients were hospitalized on multiple occasions within 90 days of the first positive COVID-19 test and therefore were excluded. This was because multiple hospitalizations within a short timeframe introduce another variable, thereby complicating the analysis, and making it difficult to assess outcomes due to the initial COVID-19 infection. Additionally, thirteen patients were not hospitalized within 14 days of their initial positive test but were then hospitalized during another positive COVID-19 test more than 14 days after the first. These patients were also excluded because delayed hospitalization may be influenced by factors other than the initial COVID-19 infection. Among the patients that were hospitalized, this study focused on those hospitalized within 14 days of the first positive test to maintain consistency and better attribute patient outcomes to the immediate impact of the initial infection. However, patients with incidental findings of COVID-19 were included in the study to ensure a comprehensive representation of the patient population.
In the working sample, many patients had multiple COVID-19 tests completed; however, only the first positive test was considered in this study. This applies to patients who had multiple COVID-19 tests within 14 days before or after a single hospitalization and those who had positive COVID-19 tests after being discharged, where only the first one was considered since the other COVID-19 tests were within 90 days of the initial COVID-19 test. Additionally, some patients who were hospitalized once within 14 days of their first positive COVID-19 test had many subsequent tests within 90 days after discharge that were also not considered.
Figure 1 also shows how the analytical groups were formed. This study conducted separate analyses based on whether patients died within 30 days of their first recorded positive COVID-19 test, and the highest level of care required, including self-management without hospitalization, admission to hospital care, and ICU admission. We also formed composite variables accounting for patients who had at least one of the specified pre-existing cardiovascular comorbidities and patients who survived beyond 30 days and who developed at least one new CVD.
The primary outcome measure will be outcomes of COVID-19 patients with and without pre-existing cardiovascular comorbidities in terms of hospital stay, ICU stay, or mortality. The secondary outcome measure will be the incidence of new cardiovascular conditions in patients with pre-existing cardiovascular comorbidities. To assess the more long-term implications of at least one COVID-19 infection, we looked at the development of new cardiac conditions up until December 2023.
The study was conducted in accordance with the Declaration of Helsinki (as revised in 2013). The study was approved by the Institutional Review Board of TCG (IRB00000918) and individual consent for this retrospective analysis was waived.
Statistical analysis
Continuous variables were reported via mean and standard deviation while categorical variables were presented as frequencies and percentages. Comparisons between groups were made with Pearson’s Chi-square tests and Mann-Whitney U tests as appropriate. Univariate and multivariate binary logistic regressions were performed to calculate the crude and adjusted odds ratios (aORs) for the 30-day mortality rate and development of new cardiac conditions. We used three models to depict ORs. Model 1 is the unadjusted OR, Model 2 adjusts for age and gender, and Model 3 adjusts for age, gender, and having at least one comorbidity. All ORs will be presented with their corresponding 95% confidence interval (CI) and P value. Model goodness of fit was assessed with the Hosmer-Lemeshow test and all reported ORs met test criteria. All statistical analysis was performed using IBM SPSS (Version 29.0.0.0). Results were considered statistically significant if the two-sided P value was <0.050.
Results
A total of 4,688 patients met the inclusion criteria for the study. Females accounted for 56.5% (n=2,651) of the sample, and males made up the remaining 43.5% (n=2,037). The median age was 53.3 years, and the cohort was 95.8% non-Hispanic individuals (Table 2).
Table 2
Demographic variable | All patients (n=4,688) | Pre-existing condition | Newly developed condition | |||||
---|---|---|---|---|---|---|---|---|
No (n=2,274) | Yes (n=2,414) | P value | No (n=3,593) | Yes (n=948) | P value | |||
Age (years) | 53.34 [20.3] | 41.97 [17.3] | 62.93 [17.4] | <0.001 | 49.24 [19.6] | 61.93 [17.9] | <0.001 | |
18–39 | 753 (16.1) | 683 (30.0) | 70 (2.9) | <0.001 | 714 (19.9) | 39 (4.1) | <0.001 | |
40–59 | 1,250 (26.7) | 862 (37.9) | 388 (16.1) | 1,079 (30.0) | 171 (18.0) | |||
60+ | 2,685 (57.3) | 729 (32.1) | 1,956 (81.0) | 1,800 (50.1) | 738 (77.8) | |||
Gender | <0.001 | 0.03 | ||||||
Male | 2,037 (43.5) | 902 (39.7) | 1,135 (47.0) | 1,519 (42.3) | 439 (46.3) | |||
Female | 2,651 (56.5) | 1,372 (60.3) | 1,279 (53.0) | 2,074 (57.7) | 509 (57.3) | |||
Ethnicity | <0.001 | 0.39 | ||||||
Non-Hispanic | 4,490 (95.8) | 2,118 (93.1) | 2,372 (98.3) | 3,434 (95.6) | 915 (96.5) | |||
Hispanic | 67 (1.4) | 48 (2.1) | 19 (0.8) | 56 (1.6) | 10 (1.1) | |||
Unknown | 131 (2.8) | 108 (4.7) | 23 (1.0) | 103 (2.9) | 23 (2.4) | |||
Mortality within 30 days | 147 (3.1) | 18 (0.8) | 129 (5.3) | <0.001 | – | – | – | |
Have at least one comorbidity | 2,414 (51.5) | – | – | – | 1,702 (47.4) | 583 (61.5) | <0.001 |
Data are presented as mean [SD] or n (%). SD, standard deviation.
Overall, 3.1% (n=147) of patients died within 30 days of a positive COVID-19 test. We considered the mortality rate at 30 days to allow for a comprehensive assessment of short-term outcomes during a period when patients are most susceptible to complications and mortality. We then grouped the patients by age: 18–39, 40–59, and 60+ years. These age groups were chosen to reflect the differing risks for developing cardiovascular factors between those in different stages of life. Further, these groupings also align with those used in existing literature. Our analysis showed a significant relationship between age group and death within 30 days (P<0.001). There were no deaths within 30 days in the first two age groups; however, 5.5% (n=147) of individuals in the 60+ age group died within 30 days of a positive COVID-19 test. Gender was also shown to have a significant correlation with the mortality rate (P=0.01). Specifically, there were more males in the 30-day morality group as opposed to females (53.7% vs. 46.3%, respectively) (Table 3). Overall, 3.9% of men died within 30 days while only 2.6% of women died.
Table 3
Demographic variable | Level of care | Death within 30 days | ||||||
---|---|---|---|---|---|---|---|---|
Self-managed (n=3,967) | Hospital (n=574) | ICU (n=147) | P value | No (n=4,541) | Yes (n=147) | P value | ||
Age (years) | 50.37 [19.55] | 69.16 [16.55] | 71.82 [13.07] | <0.001 | 52.49 [19.95] | 79.64 [9.818] | <0.001 | |
18–39 | 729 (18.4) | 23 (4.0) | 1 (0.7) | <0.001 | 753 (16.6) | 0 | <0.001 | |
40–59 | 1,192 (30.0) | 50 (8.7) | 8 (5.4) | 1,250 (27.5) | 0 | |||
60+ | 2,046 (51.6) | 501 (87.3) | 138 (93.9) | 2,538 (55.9) | 147 (100.0) | |||
Gender | <0.001 | 0.01 | ||||||
Male | 1,681 (42.4) | 271 (47.2) | 85 (57.8) | 1,958 (43.1) | 79 (53.7) | |||
Female | 2,286 (57.6) | 303 (52.8) | 62 (42.2) | 2,583 (56.9) | 68 (46.3) | |||
Ethnicity | 0.049 | 0.67 | ||||||
Non-Hispanic | 3,785 (95.4) | 563 (98.1) | 142 (96.6) | 4,349 (95.8) | 141 (95.9) | |||
Hispanic | 60 (1.5) | 5 (0.9) | 2 (1.4) | 66 (1.5) | 1 (0.7) | |||
Unknown | 122 (3.1) | 6 (1.0) | 3 (2.0) | 126 (2.8) | 5 (3.4) | |||
Death within 30 days | <0.001 | |||||||
No | 3,930 (99.1) | 514 (89.5) | 97 (66.0) | – | – | – | ||
Yes | 37 (0.9) | 60 (10.5) | 50 (34.0) | – | – | – | ||
Having at least one comorbidity | <0.001 | <0.001 | ||||||
No | 2,143 (54.0) | 114 (19.9) | 17 (11.6) | 2,256 (49.7) | 18 (12.2) | |||
Yes | 1,824 (46.0) | 460 (80.1) | 130 (88.4) | 2,285 (50.3) | 129 (87.8) |
Data are presented as mean [SD] or n (%). ICU, intensive care unit; SD, standard deviation.
We sorted our sample into mutually exclusive groups according to the highest level of care received. Out of the entire sample, 84.6% (n=3,967) managed their care independently without hospitalization. Twelve point two percent (n=574) were admitted to hospital care and 3.1% (n=147) required ICU admission. An assessment of the association between the highest level of care received and the incidence of mortality within 30 days of a positive COVID-19 test yielded a significant Chi-square result (P<0.001). Specifically, as the level of care increased from self-managed, to hospital admission, to ICU admission, there was a corresponding increase in the percentage of individuals who died within 30 days (Table 3).
To assess the general relationship between having a comorbidity and our categorical variables, we aggregated individual CVD comorbidities into a composite variable that indicates if a patient had at least one comorbidity. In our sample, 51.5% (n=2,414) had at least one pre-existing cardiac condition, with hypertension (45.8%; n=2,146) and ventricular arrhythmia (12.1%; n=567) being the most common. The 30-day mortality rate was found to have a significant association with having at least one preexisting cardiac comorbidity (P<0.001). The percentage of deaths within 30 days was 0.8% for patients without comorbidities but rose to 5.3% among those with at least one comorbidity (Table 2). A Chi-square test also revealed a significant relationship between the highest level of care received and having a pre-existing cardiac condition (P<0.001). Specifically, as the highest level of care required increased, the percentage of patients that had a comorbidity increased from 46.0% to 80.1% to 88.4% (Table 3).
Mann-Whitney U tests revealed that the relationship between the length of hospital stay and death within 30 days of a positive COVID-19 test was statistically significant (P=0.03); however, it was not significant for the length of ICU stay (P=0.20). It was also shown that the association between the length of hospital stay and having a pre-existing comorbidity was statistically significant (P<0.001), but that it was not significant for the length of ICU stay (P=0.51) (Table 4).
Table 4
Patient characteristics | Length of hospital stay | Length of ICU stay | |||
---|---|---|---|---|---|
Mean (SD) | P value | Mean (SD) | P value | ||
Death within 30 days | 0.03 | 0.20 | |||
No | 7.29 (8.0) | 5.50 (8.0) | |||
Yes | 7.83 (5.9) | 5.92 (5.2) | |||
Having at least one comorbidity | <0.001 | 0.51 | |||
No | 4.99 (4.3) | 5.57 (4.4) | |||
Yes | 7.90 (8.2) | 5.66 (7.4) |
ICU, intensive care unit; SD, standard deviation.
This study also conducted univariate and multivariate binary logistic regression tests to assess the odds of mortality within 30 days with each comorbidity. Table 5 shows that the independent odds of mortality within 30 days (Model 1) were statistically significant for each comorbidity except for pericarditis and myocarditis. Model 2 shows that the adjusted odds of mortality were significant for all the comorbidities except for pericarditis, myocarditis, ischemic cardiomyopathy, and pulmonary embolism. Model 3 shows that the further adjusted odds of mortality were significant for all comorbidities except hypertension, pericarditis, myocarditis, ischemic cardiomyopathy, angina, and pulmonary embolism. The crude and aORs from all three models are depicted in Figure 2.
Table 5
Variables | Model 1 | Model 2 | Model 3 | |||||
---|---|---|---|---|---|---|---|---|
OR (95% CI) | P value | OR (95% CI) | P value | OR (95% CI) | P value | |||
Hypertension | 5.27 (3.48, 7.99) | <0.001 | 2.21 (1.45, 3.36) | <0.001 | 1.21 (0.62, 2.35) | 0.58 | ||
Atrial fibrillation | 5.79 (4.10, 8.18) | <0.001 | 3.07 (2.17, 4.36) | <0.001 | 2.57 (1.78, 3.70) | <0.001 | ||
Ventricular arrythmia | 3.63 (2.54, 5.20) | <0.001 | 2.18 (1.52, 3.13) | <0.001 | 1.77 (1.22, 2.58) | 0.003 | ||
Myocardial infarction | 4.64 (3.04, 7.07) | <0.001 | 2.54 (1.66, 3.88) | <0.001 | 2.10 (1.36, 3.23) | <0.001 | ||
Stroke | 4.07 (2.84, 5.84) | <0.001 | 2.22 (1.54, 3.19) | <0.001 | 1.81 (1.24, 2.63) | 0.002 | ||
Pericarditis | 0.97 (0.23, 3.98) | 0.96 | 0.61 (0.15, 2.53) | 0.50 | 0.50 (0.12, 2.09) | 0.34 | ||
Myocarditis | 0.00 (0.00, 0.00) | >0.99 | 0.00 (0.00, 0.00) | >0.99 | 0.00 (0.00, 0.00) | >0.99 | ||
Acute coronary artery disease | 4.82 (2.68, 8.67) | <0.001 | 2.78 (1.54, 5.02) | <0.001 | 2.31 (1.27, 4.18) | 0.006 | ||
Ischemic cardiomyopathy | 3.57 (1.82, 7.01) | <0.001 | 1.89 (0.96, 3.75) | 0.07 | 1.57 (0.79, 3.11) | 0.20 | ||
Angina | 3.07 (1.97, 4.80) | <0.001 | 1.65 (1.05, 2.59) | 0.03 | 1.34 (0.85, 2.12) | 0.21 | ||
Congestive heart failure | 7.49 (5.32, 10.53) | <0.001 | 3.98 (2.83, 5.61) | <0.001 | 3.42 (2.38, 4.91) | <0.001 | ||
Pulmonary embolism | 2.36 (1.13, 4.94) | 0.02 | 1.57 (0.75, 3.30) | 0.24 | 1.28 (0.61, 2.71) | 0.51 | ||
Deep vein thrombosis | 4.13 (2.51, 6.78) | <0.001 | 2.53 (1.53, 4.18) | <0.001 | 2.09 (1.26, 3.46) | 0.004 | ||
Having at least one comorbidity | 7.08 (4.31, 11.63) | <0.001 | 2.72 (1.65, 4.49) | <0.001 | – | – |
OR, odds ratio; CI, confidence interval.
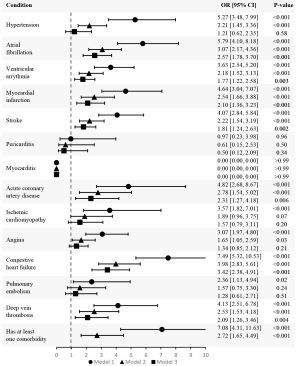
To assess the long-term implications of contracting COVID-19 at least once on the development of new cardiac conditions, we made a composite patient cohort comprised of individuals who survived the first 30 days of COVID-19 infection (n=4,541). We decided to consider beyond-30-day survival and follow-up until December 2023 to adequately capture the enduring impact of COVID-19 on cardiovascular health. This extended duration was chosen to provide valuable insight into the potential long-term cardiovascular consequences of surviving COVID-19 in the short term.
We found that 20.9% (n=948) of those who survived beyond 30 days developed at least one new cardiac condition after their initial COVID-19 positive test. This statistic includes both those who had and did not have any preexisting cardiac conditions. Of the 2,256 patients who did not have a preexisting comorbidity, 19.2% developed a new cardiac condition after their first positive COVID-19 test. Of the 2,285 patients that did have a preexisting cardiovascular condition, 32.0% developed another cardiac condition after their first positive COVID-19 test. Chi-square tests revealed that there is a significant association between having at least one comorbidity and developing a new cardiac diagnosis after COVID-19 infection in the aggregate sample of all patients who survived beyond 30 days (P<0.001) (Table 2).
A Chi-square test also revealed that there is a significant relationship between the highest level of care required and the development of a new cardiovascular disorder in those who survived the first 30 days of COVID-19 infection (P<0.001). Specifically, the percentage of people in each level of care group that developed a new cardiac condition increased as the highest level of care required increased: 16.9% of self-managed patients, 44.7% of patients admitted to the hospital, and 54.6% of patients under ICU care developed a new cardiac diagnosis (Figure 3).
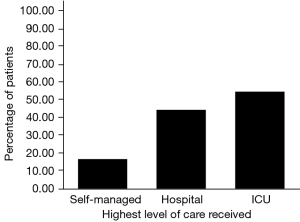
To evaluate the odds of developing each CVD after at least one COVID-19 infection according to the highest level of care required, we conducted two different analyses. In the first analysis, based on Model 3, we included all the patients who survived beyond 30 days in our sample; however, the sample for the second analysis was limited to only those who had comorbidities. The second analysis was based on Model 2 because this model does not adjust for having comorbidities whereas Model 3 does. Within each of those samples, we created sub-cohorts comprised of patients who did not have a pre-existing diagnosis of each specific CVD before their COVID-19 infection date. The logistic regression analysis focused exclusively on the groups without the disease and evaluated the odds of developing said CVD according to the highest level of care received with self-managed care as the reference category. We present the aORs in Tables 6,7 for both analyses, respectively.
Table 6
Variables | Level of care | Model 3 | |
---|---|---|---|
OR (95% CI) | P value | ||
Hypertension | Hospital | 2.60 (1.75, 3.88) | <0.001 |
ICU | 3.77 (1.68, 8.43) | 0.001 | |
Atrial fibrillation | Hospital | 3.28 (2.25, 4.78) | <0.001 |
ICU | 4.26 (2.17, 8.36) | <0.001 | |
Ventricular arrythmia | Hospital | 2.59 (1.79, 3.76) | <0.001 |
ICU | 2.55 (1.22, 5.31) | 0.01 | |
Myocardial infarction | Hospital | 3.90 (2.41, 6.32) | <0.001 |
ICU | 5.09 (2.35, 11.03) | <0.001 | |
Stroke | Hospital | 3.18 (2.27, 4.47) | <0.001 |
ICU | 1.89 (0.88, 4.08) | 0.10 | |
Pericarditis | Hospital | 5.25 (2.54, 10.85) | <0.001 |
ICU | 1.57 (0.20, 12.11) | 0.67 | |
Myocarditis | Hospital | 12.29 (1.74, 86.74) | 0.01 |
ICU | 0.00 (0.00, 0.00) | >0.99 | |
Acute coronary artery disease | Hospital | 3.34 (1.59, 7.04) | 0.002 |
ICU | 1.12 (0.15, 8.59) | 0.91 | |
Ischemic cardiomyopathy | Hospital | 2.21 (0.92, 5.26) | 0.08 |
ICU | 5.34 (1.71, 16.70) | 0.004 | |
Angina | Hospital | 1.61 (0.78, 3.33) | 0.20 |
ICU | 0.70 (0.09, 5.24) | 0.73 | |
Congestive heart failure | Hospital | 3.84 (2.68, 5.52) | <0.001 |
ICU | 2.86 (1.35, 6.04) | 0.006 | |
Pulmonary embolism | Hospital | 4.05 (2.28, 7.19) | <0.001 |
ICU | 4.51 (1.68, 12.14) | 0.003 | |
Deep vein thrombosis | Hospital | 3.09 (1.74, 5.49) | <0.001 |
ICU | 4.53 (1.82, 11.26) | 0.001 |
OR, odds ratio; CI, confidence interval; ICU, intensive care unit.
Based on Model 3 (Table 6; Figure 4), the odds of developing ischemic cardiomyopathy or angina were not statistically significant in hospitalized patients. Similarly, for patients under ICU care, the odds of developing stroke, pericarditis, myocarditis, acute coronary artery disease, and angina were not statistically significant. Further, across all the diseases we assessed, except for stroke, pericarditis, acute coronary artery disease, and angina, the odds of developing the condition increased as the level of care increased from hospital care to ICU care.
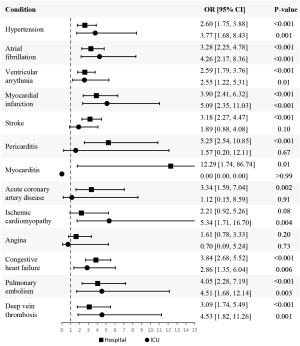
In the second analysis (Table 7; Figure 5), we found that the odds of developing myocarditis, ischemic cardiomyopathy, and angina were not statistically significant in hospitalized patients. For patients admitted to the ICU, the odds of developing hypertension, stroke, pericarditis, myocarditis, acute coronary artery disease, and angina were not statistically significant. The odds of developing each condition increased as the level of care increased for all the conditions we assessed except for hypertension, stroke, pericarditis, myocarditis, acute coronary artery disease, and angina.
Table 7
Variables | Level of care | Model 2 | |
---|---|---|---|
OR (95% CI) | P value | ||
Hypertension | Hospital | 2.47 (1.14, 5.35) | 0.02 |
ICU | 1.38 (0.44, 4.31) | 0.58 | |
Atrial fibrillation | Hospital | 3.18 (2.10, 4.82) | <0.001 |
ICU | 4.62 (2.31, 9.24) | <0.001 | |
Ventricular arrythmia | Hospital | 2.26 (1.49, 3.4) | <0.001 |
ICU | 2.33 (1.06, 5.09) | 0.03 | |
Myocardial infarction | Hospital | 3.98 (2.35, 6.77) | <0.001 |
ICU | 5.81 (2.63, 12.84) | <0.001 | |
Stroke | Hospital | 2.93 (2.00, 4.28) | <0.001 |
ICU | 2.03 (0.93, 4.43) | 0.07 | |
Pericarditis | Hospital | 5.69 (2.61, 12.37) | <0.001 |
ICU | 1.79 (0.23, 14.04) | 0.58 | |
Myocarditis | Hospital | 7.65 (0.95, 61.81) | 0.06 |
ICU | 0.00 (0.00, 0.00) | >0.99 | |
Acute coronary artery disease | Hospital | 3.39 (1.60, 7.17) | 0.001 |
ICU | 1.14 (0.15, 8.79) | 0.90 | |
Ischemic cardiomyopathy | Hospital | 1.99 (0.79, 5.05) | 0.15 |
ICU | 5.31 (1.68, 16.81) | 0.005 | |
Angina | Hospital | 1.43 (0.67, 3.07) | 0.36 |
ICU | 0.68 (0.09, 5.09) | 0.71 | |
Congestive heart failure | Hospital | 3.35 (2.28, 4.92) | <0.001 |
ICU | 2.89 (1.36, 6.17) | 0.006 | |
Pulmonary embolism | Hospital | 3.49 (1.86, 6.52) | <0.001 |
ICU | 4.53 (1.66, 12.37) | 0.003 | |
Deep vein thrombosis | Hospital | 2.62 (1.38, 4.96) | 0.003 |
ICU | 4.65 (1.84, 11.75) | 0.001 |
OR, odds ratio; CI, confidence interval; ICU, intensive care unit.
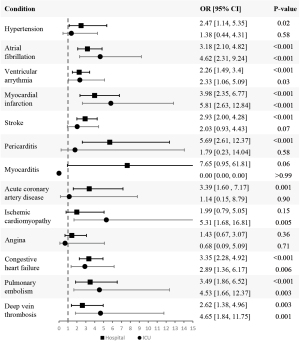
We did not adjust for smoking status within our analyses due to the limitations of conducting a retrospective study. As a result of discrepancies in our data report, we noted that smoking status may not have been consistently and uniformly documented for patients in the study population. Due to our inability to prospectively collect smoking data, we opted to not adjust for smoking status to avoid introducing inaccuracies due to potentially incomplete data. Therefore, we present the amount and percentage of patients of each smoking status for each analytical group in Table 8. Overall, 62.4% (n=2,924) of the study sample were non-smokers; 32.8% (n=1,538) were former smokers, and 4.8% (n=226) were smokers at the time of their initial positive COVID-19 test. Chi-square tests revealed a significant association (P<0.001) between smoking status and the highest level of care required, having a pre-existing comorbidity, and death within 30 days. In those that survived beyond 30 days, Chi-square tests also revealed a significant association between smoking status and having a new CVD diagnosis in the entire sample (P<0.001). This association was also held in samples of only patients without pre-existing CVD (P<0.001) and only patients with pre-existing CVD (P<0.001) (Table 8).
Table 8
Variables | Smoking status, n (%) | |||
---|---|---|---|---|
Non-smoker | Former smoker | Current smoker | P value | |
Overall (n=4,688) | 2,924 (62.4) | 1,538 (32.8) | 226 (4.8) | – |
Level of care | <0.001 | |||
Self-managed | 2,569 (64.8) | 1,187 (29.9) | 211 (5.3) | |
Hospital | 284 (49.5) | 277 (48.3) | 13 (2.3) | |
ICU | 71 (48.3) | 74 (50.3) | 2 (1.4) | |
Has a pre-existing comorbidity | <0.001 | |||
No | 1,647 (72.4) | 500 (22.0) | 127 (5.6) | |
Yes | 1,277 (52.9) | 1,038 (43.0) | 99 (4.1) | |
Died within 30 days | <0.001 | |||
No | 2,853 (62.8) | 1,463 (32.2) | 225 (5.0) | |
Yes | 71 (48.3) | 75 (51.0) | 1 (0.7) | |
Patients who survived beyond 30 days who received a new cardiac diagnosis | ||||
Total (n=4,541) | 2,853 (62.8) | 1,463 (32.2) | 225 (5.0) | – |
No | 2,335 (65.0) | 1,097 (30.5) | 161 (4.5) | <0.001 |
Yes | 518 (54.6) | 366 (38.6) | 64 (6.8) | |
Patients surviving past 30 days without pre-existing CVD who received a new cardiac diagnosis | ||||
Total (n=2,256) | 1,632 (72.3) | 497 (22.0) | 127 (5.6) | – |
No | 1,345 (73.8) | 388 (21.3) | 89 (4.9) | <0.001 |
Yes | 287 (66.1) | 109 (25.1) | 38 (8.8) | |
Patients surviving past 30 days with at least one pre-existing CVD who received a new cardiac diagnosis | ||||
Total (n=2,285) | 1,221 (53.4) | 966 (42.3) | 98 (4.3) | – |
No | 872 (56.1) | 624 (40.2) | 58 (3.7) | <0.001 |
Yes | 349 (47.7) | 342 (46.8) | 40 (5.5) |
ICU, intensive care unit; CVD, cardiovascular disease.
Discussion
Our findings provide valuable insights into the impact of COVID-19 on those with pre-existing cardiac comorbidities. Having cardiac comorbidities has been shown to result in worse disease outcomes including higher mortality rates, requiring higher levels of care, longer hospital stays, and the development of new cardiac conditions.
Overall, 3.1% of patients died within 30 days of a positive COVID-19 test. Our results show that more males died within 30 days which is consistent with many studies which report that males have a higher chance of death (17).
Examination of the age distribution revealed that all the patients who died were in the ‘60+’ age group. Those in older age groups were also more likely to have a pre-existing comorbidity (P<0.001). Many studies also show that the mortality rate was highest for patients in the 60+ age group (2,18). As such, the elderly have therefore been identified as a vulnerable population for COVID-19 infection because they are more likely to have comorbidities and compromised immune systems (19).
This study revealed that the mortality rate among those with at least one cardiac comorbidity was higher at 5.3% compared to the 0.8% of people without a comorbidity. This aligns with existing literature suggesting that COVID-19-positive individuals with cardiac comorbidities are more vulnerable to mortality (20,21). A report of 72,314 COVID-19 cases from the Chinese Center for Disease Control and Prevention showed that the overall fatality rate of COVID-19 was 2.3%, but it increased to 10.5% in individuals with pre-existing CVD (22). In line with our study, studies from Indonesia and the UK corroborate that hypertension was the most common comorbid condition (18,23). We noted that a significant elevated risk of 30-day mortality was associated with hypertension in Model 1 and 2 but not in Model 3 (Model 1: OR =5.27, 95% CI: 3.48, 7.99, P<0.001; Model 2: OR =2.21, 95% CI: 1.45, 3.36, P<0.001; Model 3: OR =1.21, 95% CI: 0.62, 2.35, P=0.58) (Figure 2; Table 5). A study from Australia also reported in a univariate analysis that hypertension was associated with higher odds of in-hospital mortality (OR =3.22, 95% CI: 2.30, 4.50, P<0.001), but they did not note a significant association in their adjusted model (24). They did find, however, that pre-existing CVD in general was associated with higher odds of in-hospital mortality in both their univariate and multivariate models (OR =5.10, 95% CI: 3.68, 7.06, P<0.001; aOR =1.76, 95% CI: 1.21, 2.55, P=0.003) (24).
Notably, our results also showed that generally having at least one cardiac comorbidity is associated with higher odds of death (Model 1: OR =7.08, 95% CI: 4.31, 11.63, P<0.001; Model 2: OR =2.72, 95% CI: 1.65, 4.49, P<0.001) (Figure 2; Table 5). Although COVID-19 primarily affects the respiratory system, the virus enters cells through angiotensin-converting enzyme 2 (ACE2), which is heavily expressed in the heart (25). ACE2 is a key component of the renin-angiotensin-aldosterone system (RAAS) which regulates cardiovascular function, but virus entry leads to downregulation of ACE2, thereby dysregulating RAAS, contributing to cardiovascular breakdown. These abnormalities in RAAS can exacerbate the underlying cardiovascular dysfunction already present from the pre-existing cardiovascular comorbidities. This combination can lead to worse outcomes and an increased risk of mortality due to increased cardiovascular vulnerability. Moreover, COVID-19 infection triggers a cytokine storm which leads to further damage to the heart, further amplifying the risk of adverse outcomes (26). Additionally, with CVDs being the most common cause of death from non-communicable diseases worldwide, they are also known to be associated with having a high level of comorbidities. A cross-sectional study in the Netherlands noted that one CVD increased the risk of another CVD comorbidity and the occurrence of other chronic diseases (27). All these factors may have a compounding effect on mortality risk.
The highest odds of 30-day mortality were noted for patients with CHF (Model 1: OR =7.49, 95% CI: 5.32, 10.53, P<0.001; Model 2: OR =3.98, 95% CI: 2.83, 5.61, P<0.001; Model 3: OR =3.42, 95% CI: 2.38, 4.91, P<0.001) (Figure 2; Table 5). A review of 26 papers identified that heart failure is an independent predictor of mortality in hospitalized COVID-19 patients (28). It is important to note that all of those included studies only used hospitalized patients in their sample whereas this study also includes non-hospitalized patients in the sample.
Overall, adjusting for age and sex and further adjusting for having at least one comorbidity in each of the pre-existing conditions attenuated the odds of mortality. A study by Vasbinder et al. also noted attenuation after adjusting for demographics and further attenuation after adjusting for comorbidities (20). A limitation of this study is that we do not have a model adjusted for each individual comorbidity, rather, we used a composite variable. We were unable to report such findings because the individual comorbidity-adjusted model that we tried did not pass the Hosmer-Lemeshow goodness-of-fit test indicating that any results would not have been accurately represented. The attenuation that Vasbinder et al. noted suggests that demographics and the few individual comorbidities they adjusted for, some non-cardiac in nature, influence the relationship between each pre-existing CVD and the 30-day mortality rate (20). It is important to acknowledge that although our study may provide valuable insights toward the odds of death in CVD patients, our findings should be interpreted with caution since we did not adjust for individual comorbidities or non-cardiac comorbidities which are known to influence the association.
Interestingly, death within 30 days and having a comorbidity was significantly associated with the length of hospital stay but was not significantly associated with the length of ICU stay. A likely explanation for the lack of significance associated with the length of ICU stay is that the impact of having comorbidities may be overshadowed by the severity of the patient’s case. Moreover, patients in the ICU are receiving immediate specialized care to stabilize patients and prevent any further deterioration which may mitigate the impact of the length of ICU stay on the risk of 30-day mortality. The impact of having comorbidities has, however, been repeatedly shown to be significantly associated with the length of hospital stay in many studies (29).
Our results also convey a significant relationship between having a comorbidity and the incidence of at least one new cardiac condition. A study using healthcare databases from the US Department of Veterans Affairs by Xie et al. showed that veterans who survived beyond the first 30 days after COVID-19 infection were at an increased risk of CVDs such as cerebrovascular disorders, dysrhythmias, ischemic and non-ischemic heart disease, pericarditis, myocarditis, heart failure, and thromboembolic disease (15). They also elucidated that these risks prevailed even in those who were not hospitalized during the first 30 days following infection and that their risks increased as the level of care they received increased (self-managed recovery, hospitalized care, and ICU care) (15). They noted an increasing risk in all assessed disorders except for angina. Increasing odds of developing a new cardiac condition can also be noted in our results although not for all the diseases we assessed. In our first logistic regression analysis assessing the odds of developing a new cardiac condition according to the level of care including all patients who survived beyond 30 days in the sample, based on Model 3, we noted that of the conditions where the P values for both levels of care were statistically significant, the odds of developing hypertension, atrial fibrillation, MI, pulmonary embolism, and deep vein thrombosis increased as the level of care increased. Conversely, we noted that the odds of developing ventricular arrhythmia and congestive heart failure decreased. The second analysis was similar to the first analysis, with the only difference being that the sample was restricted to only those with pre-existing comorbidities. This was based on Model 2, and we noted that the odds of developing atrial fibrillation, ventricular arrhythmia, MI, pulmonary embolism, and deep vein thrombosis increased as the level of care required increased, and the only one that decreased was congestive heart failure.
While this study doesn’t assess causality, the study by Xie et al. established that the associations between COVID-19 and cardiovascular outcomes is related to the exposure to COVID-19 itself. They utilized a contemporary control comprised of individuals who did not contract COVID-19 but were part of the same time period as the COVID-19 cases included in the study and a historical control cohort comprised of individuals who used the US Veterans Health Administration (VHA) system in 2017, a time period predating the pandemic, and did not fall into their COVID-19-infected cases group. They conducted difference-in-differences analyses relative to the contemporary and historical control groups in the pre-COVID-19 and post-COVID-19 infection periods and attained incident rate ratios significantly higher than 1 for all CVDs they assessed. This analysis showed that the increased risk of CVD following acute COVID-19 infection is in fact attributable to the COVID-19 infection itself, rather than other factors.
The lack of significance for certain associations in both of our analyses may be attributed to our sample size. In the first analysis, the number of patients who developed each of the disorders where both hospital stay and ICU stay yielded non-statistically significant ORs was fewer than 50, except for stroke. Similarly, for the second analysis, there were also fewer than 50 patients who developed each of the disorders except for stroke and hypertension. An insufficient sample size may have compromised the ability to identify the association between the level of care and the development of those disorders.
This study also noted significant associations between smoking status and the various analytical groups depicted in Table 8. While we did not conduct logistic regression analyses involving smoking status due to incomplete or inconsistent documentation, a study by Gallus et al. reports that current smokers had a significantly increased risk of COVID-19 mortality (aOR =2.17, 95% CI: 1.06, 4.41), adjusted for sex, age, and level of education, when compared to non-smokers (30). A study by Poudel et al., which focused on the association between smoking and patient outcomes in patients hospitalized due to COVID-19 infection, noted an increased risk of death (aOR =1.41; 95% CI: 1.21, 1.64), mechanical ventilation use (aOR =1.15; 95% CI: 1.01, 1.32), and major adverse cardiovascular events (aOR =1.27; 95% CI: 1.05, 1.52) among current smokers compared to non-smokers after adjusting for sociodemographic characteristics, medical history, medication use, and the time of hospital admission (31). These results highlight the impact of tobacco usage on patient outcomes, especially in light of the COVID-19 pandemic.
Limitations and future studies
While this study makes notable findings, it also poses some limitations. Firstly, it is important to note that this study relied on existing medical records, and the retrospective nature of this study may impact the quality of the data we collected. The Epic data report only populates mortality status if the patient died within our facility or if the clinic is notified; patients who died at home may not be accurately represented. Additionally, this study gathered patients from the 2020 calendar year, but the various new strains that have emerged since then could impact the generalizability of these results. As the virus evolves, specific variants of concern arise which impact various aspects of disease progression such as increased transmissibility, susceptibility to reinfection, evasion of neutralizing antibodies, and enhanced binding to the human ACE2 receptor (32). Future studies should investigate the impact of different variants and explore the cardiovascular outcomes associated with certain variants. Another limitation of this study is that it considered incidental findings of COVID-19 which may be related to milder cases, potentially skewing the sample. We also only had a small cohort of patients who developed some of the disorders mentioned, impacting the significance of the ORs. This study also did not adjust for various other comorbidities or smoking status in the logistic regression models. Additionally, we did not ascertain vaccination status which may affect the risk of subsequent COVID-19 infection after the data collection period and therefore affect the development of comorbidities. Our models do not account for the potential influence of smoking status, other individual comorbidities, or vaccination status on patient outcomes which is something future studies should consider in a similar population. Future studies should also consider how pre-existing CVD impacts the incidence of other diseases affecting other major body systems.
Conclusions
This study sheds light on the interplay between cardiac comorbidities and disease severity in terms of short-term mortality, length of and highest level of care received, and also focuses on the development of new cardiac disorders within the rural population of TGC. Since CVD is the leading cause of death worldwide, it is important that we understand the correlation between such a prevalent group of disorders and the COVID-19 virus. This study emphasized previous findings, particularly that having cardiac comorbidities results in higher mortality rates, higher levels of required care, longer hospital stays, and higher odds of developing certain new CVDs in this population. We hope that healthcare professionals can better tailor treatment paradigms and allocate resources effectively to mitigate the impact of COVID-19 on patients with pre-existing cardiac conditions to ensure the best patient outcomes.
Acknowledgments
The authors would like to thank the clinical data analyst, Jeffery Dorman, for gathering and retrieving the pertinent patient data from EPIC and the Institutional Review Board of the Guthrie Clinic for approving this project. The authors also thank Vicky Hickey for research guidance.
Funding: None.
Footnote
Provenance and Peer Review: This article was commissioned by the editorial office, Journal of Public Health and Emergency for the series “Primary Care and Public Health in the Wake of the COVID-19 Pandemic”. The article has undergone external peer review.
Reporting Checklist: The authors have completed the STROBE reporting checklist. Available at https://jphe.amegroups.com/article/view/10.21037/jphe-24-22/rc
Data Sharing Statement: Available at https://jphe.amegroups.com/article/view/10.21037/jphe-24-22/dss
Peer Review File: Available at https://jphe.amegroups.com/article/view/10.21037/jphe-24-22/prf
Conflicts of Interest: Both authors have completed the ICMJE uniform disclosure form (available at https://jphe.amegroups.com/article/view/10.21037/jphe-24-22/coif). The series “Primary Care and Public Health in the Wake of the COVID-19 Pandemic” was commissioned by the editorial office without any funding or sponsorship. V.O.K. served as an unpaid Guest Editor of the series and serves as an unpaid editorial board member of the Journal of Public Health and Emergency from April 2023 to March 2025. The authors have no other conflicts of interest to declare.
Ethical Statement: The authors are accountable for all aspects of the work in ensuring that questions related to the accuracy or integrity of any part of the work are appropriately investigated and resolved. The study was conducted in accordance with the Declaration of Helsinki (as revised in 2013). The study was approved by the Institutional Review Board of The Guthrie Clinic (IRB00000918) and individual consent for this retrospective analysis was waived.
Open Access Statement: This is an Open Access article distributed in accordance with the Creative Commons Attribution-NonCommercial-NoDerivs 4.0 International License (CC BY-NC-ND 4.0), which permits the non-commercial replication and distribution of the article with the strict proviso that no changes or edits are made and the original work is properly cited (including links to both the formal publication through the relevant DOI and the license). See: https://creativecommons.org/licenses/by-nc-nd/4.0/.
References
- WHO Coronavirus (COVID-19) Dashboard [Internet] [cited 2023 Oct 20]. Available online: https://covid19.who.int
- Djaharuddin I, Munawwarah S, Nurulita A, et al. Comorbidities and mortality in COVID-19 patients. Gac Sanit 2021;35:S530-2. [Crossref] [PubMed]
- Kompaniyets L, Pennington AF, Goodman AB, et al. Underlying Medical Conditions and Severe Illness Among 540,667 Adults Hospitalized With COVID-19, March 2020–March 2021. Prev Chronic Dis 2021;18:E66. [Crossref] [PubMed]
- Bajgain KT, Badal S, Bajgain BB, et al. Prevalence of comorbidities among individuals with COVID-19: A rapid review of current literature. Am J Infect Control 2021;49:238-46. [Crossref] [PubMed]
- Dou Q, Wei X, Zhou K, et al. Cardiovascular Manifestations and Mechanisms in Patients with COVID-19. Trends Endocrinol Metab 2020;31:893-904. [Crossref] [PubMed]
- Chakinala RC, Shah CD, Rakholiya JH, et al. COVID-19 Outcomes Amongst Patients With Pre-existing Cardiovascular Disease and Hypertension. Cureus 2021;13:e13420. [Crossref] [PubMed]
- Peng M, He J, Xue Y, et al. Role of Hypertension on the Severity of COVID-19: A Review. J Cardiovasc Pharmacol 2021;78:e648-55. [Crossref] [PubMed]
- Standl E, Schnell O. Heart failure outcomes and Covid-19. Diabetes Res Clin Pract 2021;175:108794. [Crossref] [PubMed]
- Kerolos MM, Ruge M, Gill A, et al. Clinical outcomes of COVID-19 infection in patients with pre-existing cardiovascular disease. Am Heart J Plus 2022;20:100189. [Crossref] [PubMed]
- Khawaja SA, Mohan P, Jabbour R, et al. COVID-19 and its impact on the cardiovascular system. Open Heart 2021;8:e001472. [Crossref] [PubMed]
- Szarpak L, Mierzejewska M, Jurek J, et al. Effect of Coronary Artery Disease on COVID-19-Prognosis and Risk Assessment: A Systematic Review and Meta-Analysis. Biology (Basel) 2022;11:221. [Crossref] [PubMed]
- López-Pérez CA, Santa Cruz-Pavlovich FJ, Montiel-Cortés JE, et al. Risk Factors for Mortality of Hospitalized Adult Patients with COVID-19 Pneumonia: A Two-Year Cohort Study in a Private Tertiary Care Center in Mexico. Int J Environ Res Public Health 2023;20:4450. [Crossref] [PubMed]
- Pachpande V, Senapathi SHV, Williams K, et al. Demographics, comorbidities, and laboratory parameters in hospitalized patients with SARS-CoV2 infection at a community hospital in rural Pennsylvania. PLoS One 2022;17:e0267468. [Crossref] [PubMed]
- Wang W, Wang CY, Wang SI, et al. Long-term cardiovascular outcomes in COVID-19 survivors among non-vaccinated population: A retrospective cohort study from the TriNetX US collaborative networks. EClinicalMedicine 2022;53:101619. [Crossref] [PubMed]
- Xie Y, Xu E, Bowe B, et al. Long-term cardiovascular outcomes of COVID-19. Nat Med 2022;28:583-90. [Crossref] [PubMed]
- Toro-Huamanchumo CJ, Hilario-Gomez MM, Pinedo-Castillo L, et al. Clinical and epidemiological features of patients with COVID-19 reinfection: a systematic review. New Microbes New Infect 2022;48:101021. [Crossref] [PubMed]
- Fabião J, Sassi B, Pedrollo EF, et al. Why do men have worse COVID-19-related outcomes? A systematic review and meta-analysis with sex adjusted for age. Braz J Med Biol Res 2022;55:e11711. [Crossref] [PubMed]
- Karyono DR, Wicaksana AL. Current prevalence, characteristics, and comorbidities of patients with COVID-19 in Indonesia. Journal of Community Empowerment for Health 2020;3:77. [Crossref]
- Damayanthi HDWT, Prabani KIP, Weerasekara I. Factors Associated for Mortality of Older People With COVID 19: A Systematic Review and Meta-analysis. Gerontol Geriatr Med 2021;7:23337214211057392. [Crossref] [PubMed]
- Vasbinder A, Meloche C, Azam TU, et al. Relationship Between Preexisting Cardiovascular Disease and Death and Cardiovascular Outcomes in Critically Ill Patients With COVID-19. Circ Cardiovasc Qual Outcomes 2022;15:e008942. [Crossref] [PubMed]
- Dessie ZG, Zewotir T. Mortality-related risk factors of COVID-19: a systematic review and meta-analysis of 42 studies and 423,117 patients. BMC Infect Dis 2021;21:855. [Crossref] [PubMed]
- Wu Z, McGoogan JM. Characteristics of and Important Lessons From the Coronavirus Disease 2019 (COVID-19) Outbreak in China: Summary of a Report of 72 314 Cases From the Chinese Center for Disease Control and Prevention. JAMA 2020;323:1239-42. [Crossref] [PubMed]
- Patel R, Kooner JS, Zhang W. Comorbidities associated with the severity of COVID-19, and differences across ethnic groups: a UK Biobank cohort study. BMC Public Health 2023;23:1566. [Crossref] [PubMed]
- Sritharan HP, Bhatia KS, van Gaal W, et al. Association between pre-existing cardiovascular disease, mortality and cardiovascular outcomes in hospitalised patients with COVID-19. Front Cardiovasc Med 2023;10:1224886. [Crossref] [PubMed]
- Zou X, Chen K, Zou J, et al. Single-cell RNA-seq data analysis on the receptor ACE2 expression reveals the potential risk of different human organs vulnerable to 2019-nCoV infection. Front Med 2020;14:185-92. [Crossref] [PubMed]
- Yu WL, Toh HS, Liao CT, et al. Cardiovascular Complications of COVID-19 and Associated Concerns: A Review. Acta Cardiol Sin 2021;37:9-17. [PubMed]
- Kendir C, van den Akker M, Vos R, et al. Cardiovascular disease patients have increased risk for comorbidity: A cross-sectional study in the Netherlands. Eur J Gen Pract 2018;24:45-50. [Crossref] [PubMed]
- John KJ, Mishra AK, Ramasamy C, et al. Heart failure in COVID-19 patients: Critical care experience. World J Virol 2022;11:1-19. [Crossref] [PubMed]
- Aghdam BH, Seyedbaglou ZK, Akhtari AS. Comparison of Mortality, Length of Stay, and Hospitalization Costs of Hospitalized COVID-19 Patients with Cardiac and Non-Cardiac Disease. Open Journal of Emergency Medicine 2023;11:57-67. [Crossref]
- Gallus S, Bosetti C, Gorini G, et al. The Association of Tobacco Smoking, Second-hand Smoke, and Novel Tobacco Products With COVID-19 Severity and Mortality in Italy: Results From the COSMO-IT Study. J Epidemiol 2023;33:367-71. [Crossref] [PubMed]
- Poudel R, Daniels LB, DeFilippis AP, et al. Smoking is associated with increased risk of cardiovascular events, disease severity, and mortality among patients hospitalized for SARS-CoV-2 infections. PLoS One 2022;17:e0270763. [Crossref] [PubMed]
- Zella D, Giovanetti M, Cella E, et al. The importance of genomic analysis in cracking the coronavirus pandemic. Expert Rev Mol Diagn 2021;21:547-62. [Crossref] [PubMed]
Cite this article as: John G, Kolade VO. Outcomes and development of new cardiovascular conditions in patients with pre-existing cardiovascular disease after contracting COVID-19. J Public Health Emerg 2025;9:1.