Fitness and strength in young adulthood and protection from future heart failure
We are in the midst of a heart failure (HF) pandemic with wide-ranging implications for aging populations, physicians, and healthcare delivery systems (1). The major epidemiologic determinants for HF have been traditionally viewed as hypertension, coronary artery disease, valvular disease, and more recently obesity, diabetes, and kidney disease (2,3). Among those with HF, approximately half have HF with reduced ejection fraction (HFrEF) and the other half with preserved ejection fraction (HFpEF). In this issue of the European Journal of Preventive Cardiology, Lindgren and co-workers report on a ray of hope in HF epidemiology (4). They describe a protective relationship between cardiopulmonary fitness as well as muscle strength and the development of HF at a relatively young age in all categories in which it can be accounted for using automated sources of data. This report is a major advance in the cardiopulmonary fitness literature, which has had a global interest in “survival of the fittest” which was a way of explaining the relationship between fitness and mortality (5). In brief, cardiopulmonary fitness has been associated with reduced mortality not only because of improved risk factors, and modest reductions in atherosclerotic events such as myocardial infarction, but because it markedly improves the survival of potentially fatal events such as critical illness.
In the present study, the highest group of cardiopulmonary fitness (42.2% of men) was associated with a 49%, reduction in the incidence of HF after adjusting for important confounders such as age, hypertension, diabetes, congenital heart disease, alcohol abuse and measured blood pressure (model 2) (4). Likewise, in the same model, the highest muscular strength (31.3% of men) conferred a 50% reduction in HF. The two measures had collinearity so when considered together, adjustment bias reduced the risk relationships, but does not take away from the key findings: fitness in young adulthood is associated with sharply lower rates of HF decades later.
Given the very large sample size and the internal consistency of the data, we believe the relationships reported are valid. This accepted, one must consider an array of mechanisms with the understanding that there is a large gap in time between the fitness measurements and the outcome of HF, which occurred at a young age given the time window available in the data. While at age 18 the body mass index was 22 kg/m2, none of the fitness groups appeared to be on a significant obesity trajectory at this snapshot in time (6). It is possible that the lower fitness and strength men, with lower measures of intellectual capacity, were more likely to be on a pathway towards obesity, hypertension, diabetes, and poorer health status. If exercise patterns persisted several years or decades into life, then the cardiometabolic effects could have clearly played a role. A recent analysis from the Multi-Ethnic Study of Atherosclerosis and Cardiovascular Health Study found that voluntary, leisure time physical activity in earlier life was associated with an adjusted ~25% reduction in the risk of future HF (7). The protective effect appeared to be more pronounced for HFpEF. It is known that exercise decreases body weight, improves lipids (decreasing triglycerides and increasing high-density lipoprotein cholesterol) and improves insulin resistance (decreasing fasting insulin and hemoglobin A1C). Exercise has also been shown to decrease levels of interleukin-18 and lower levels of leptin, fibrinogen, and angiotensin II. Individuals with type 2 diabetes, hypertension, dyslipidemia, or metabolic syndrome benefit more from exercise (8). Accordingly, cardiopulmonary fitness is inversely associated with fasting serum insulin, waist-to-hip ratio, and coronary heart disease (9). Obesity is associated with cardiac adiposity, characterized by the accumulation of triglyceride in the myocardium and also deposition of fat around the heart and vessels, which may lead to left ventricular hypertrophy and dysfunction. This lipid accumulation is associated with the release of proinflammatory and proatherogenic cytokines resulting in systemic or local inflammation (10). Thus obesity is clearly related to the development of HFrEF and to a greater extent for HFpEF. A reduction in the risk HF-related death may be an explanation of how cardiorespiratory fitness is broadly associated with reduced mortality among all weight groups (11). The authors may be able to further evaluate this “cardiometabolic” hypothesis by reporting BMI, hypertension, and diabetes at the time of the index hospitalization and indicate whether HF cases are HFpEF or HFrEF.
Another potential mechanism is more directly focused on the myocardium itself. It is possible cardiopulmonary fitness as reflected by peak oxygen consumption in mL/kg/min has a legacy effect in life. Simply said, stronger hearts at younger ages may remain stronger than average over the course of time and be protected from the development of HF due to an array of etiologies. Fitness and strength in young adulthood may be related to a greater proclivity towards more leisure time physical activity in later life (7). As shown in Figure 1 there is an exercise “gene set” that is activated with exercise and results in fundamental changes in cardiomyocyte development and growth, performance, and potentially long-term organ function involving an array of cellular and tissue factors (12). Exercise induces cardiac expression of insulin-like growth factor-1, which activates the PI3K-Akt cascade resulting in cardiomyocyte hypertrophy. Simultaneous inhibition of the transcription factor C/EBPβ contributes to activation of an exercise gene set (cardiac specific transcription regulators GATA 4, alfa-MHC, Mef2C) which results in expression of many structural proteins enabling cell growth and differentiation (13). Exercise upregulates sirtuins 1 and 3 (Sirt 1 and Sirt3), which are nicotinamide adenine dinucleotide-dependent deacetylases and that regulate cellular metabolism, cell growth, apoptosis, and aging. Sirt1 has pro-growth and pro-survival functions in cardiomyocytes. Sirt3 protects against oxidative stress and regulates the opening of the mitochondrial permeability transition pore, which appears to be protective against age-related cardiac dysfunction. These are among many cellular changes that occur within cardiomyocytes in response to exercise. Activation and maintenance of an exercise gene set would explain why the data were so consistent across categories of HF that emerged from the codified hospitalization data. This mechanism is worthy of intensive exploration since myocardial energetics, cardiac morphology, hemodynamics, and peripheral vascular adaptation are all known to be different in the athlete compared to the non-athlete (14). The old adage is that fitness is lost quickly with a cessation of training, but perhaps there are special enduring protective effects that are yet to be discovered. The authors may be able to shed more light on this mechanism with greater detail about the index HF hospitalization including the left ventricular ejection fraction and other measures of cardiac function, hemodynamics, and morphology.
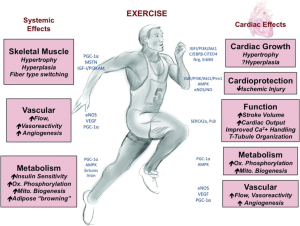
In summary, the relationship between fitness in youth and throughout life and protection from the development of HF should be hotly pursued. The mechanisms accounting for protection could lead to future diagnostic and therapeutic targets in both the heart and skeletal muscles (15). From a public health perspective, aerobic and strength conditioning programs could possibly have a large and beneficial impact given the size of the HF epidemic and its leading role in adult non-traumatic hospitalizations worldwide.
Acknowledgments
Funding: None.
Footnote
Provenance and Peer Review: This article was commissioned by the editorial office, Journal of Public Health and Emergency. The article did not undergo external peer review.
Conflicts of Interest: Both authors have completed the ICMJE uniform disclosure form (available at http://dx.doi.org/10.21037/jphe.2017.04.04). The author has no conflicts of interest to declare.
Ethical Statement: The authors are accountable for all aspects of the work in ensuring that questions related to the accuracy or integrity of any part of the work are appropriately investigated and resolved.
Open Access Statement: This is an Open Access article distributed in accordance with the Creative Commons Attribution-NonCommercial-NoDerivs 4.0 International License (CC BY-NC-ND 4.0), which permits the non-commercial replication and distribution of the article with the strict proviso that no changes or edits are made and the original work is properly cited (including links to both the formal publication through the relevant DOI and the license). See: https://creativecommons.org/licenses/by-nc-nd/4.0/.
References
- McCullough PA, Philbin EF, Spertus JA, et al. Confirmation of a heart failure epidemic: findings from the Resource Utilization Among Congestive Heart Failure (REACH) study. J Am Coll Cardiol 2002;39:60-9. [Crossref] [PubMed]
- Lloyd-Jones DM, Larson MG, Leip EP, et al. Lifetime risk for developing congestive heart failure: the Framingham Heart Study. Circulation 2002;106:3068-72. [Crossref] [PubMed]
- Yancy CW, Jessup M, Bozkurt B, et al. 2013 ACCF/AHA guideline for the management of heart failure: a report of the American College of Cardiology Foundation/American Heart Association Task Force on Practice Guidelines. J Am Coll Cardiol 2013;62:e147-239. [Crossref] [PubMed]
- Lindgren M, Åberg M, Schaufelberger M, et al. Cardiorespiratory fitness and muscle strength in late adolescence and long-term risk of early heart failure in Swedish men. Eur J Prev Cardiol 2017;2047487317689974 [Epub ahead of print]. [PubMed]
- Franklin BA. Survival of the fittest: evidence for high-risk and cardioprotective fitness levels. Curr Sports Med Rep 2002;1:257-9. [Crossref] [PubMed]
- McCullough PA, Sandberg KR, Miller WM, et al. Substantial weight gain during adulthood: the road to bariatric surgery. Prev Cardiol 2005;8:155-9. [Crossref] [PubMed]
- Pandey A, LaMonte M, Klein L, et al. Relationship Between Physical Activity, Body Mass Index, and Risk of Heart Failure. J Am Coll Cardiol 2017;69:1129-42. [Crossref] [PubMed]
- Lin X, Zhang X, Guo J, et al. Effects of Exercise Training on Cardiorespiratory Fitness and Biomarkers of Cardiometabolic Health: A Systematic Review and Meta-Analysis of Randomized Controlled Trials. J Am Heart Assoc 2015;4: [PubMed]
- Laukkanen JA, Laaksonen D, Lakka TA, et al. Determinants of cardiorespiratory fitness in men aged 42 to 60 years with and without cardiovascular disease. Am J Cardiol 2009;103:1598-604. [Crossref] [PubMed]
- Guzzardi MA, Iozzo P. Fatty heart, cardiac damage, and inflammation. Rev Diabet Stud 2011;8:403-17. [Crossref] [PubMed]
- Lee DC, Artero EG, Sui X, et al. Mortality trends in the general population: the importance of cardiorespiratory fitness. J Psychopharmacol 2010;24:27-35. [Crossref] [PubMed]
- Mann N, Rosenzweig A. Can exercise teach us how to treat heart disease? Circulation 2012;126:2625-35. [Crossref] [PubMed]
- Kojima H, Ieda M. Discovery and progress of direct cardiac reprogramming. Cell Mol Life Sci 2017; [Epub ahead of print]. [Crossref] [PubMed]
- Beaudry R, Haykowsky MJ, Baggish A, et al. A Modern Definition of the Athlete's Heart-for Research and the Clinic. Cardiol Clin 2016;34:507-14. [Crossref] [PubMed]
- Farris SD, Moussavi-Harami F, Stempien-Otero A. Heart failure with preserved ejection fraction and skeletal muscle physiology. Heart Fail Rev 2017;22:141-8. [Crossref] [PubMed]
Cite this article as: Jazi HH, McCullough PA. Fitness and strength in young adulthood and protection from future heart failure. J Public Health Emerg 2017;1:48.