Semi-quantitative microbial risk assessment: a narrative review and proposed framework for health and safety practitioners
Introduction
Exposure to pathogenic bioaerosols is ubiquitous in daily activities, regardless of lifestyle, occupation, or geographic location. Specifically, bioaerosols refer to a broad range of aerosolized particles containing biologic agents from microorganisms, plant, or animal sources (1-3). Individual bioaerosol constituents vary based on the occupational setting and may include bacteria, viruses, fungi, allergens, bacterial endotoxins, mycotoxins, β-glucans, and plant fibers (3,4). As such, a need exists to monitor and assess human health risks associated with exposure to microbial hazards, including human pathogens. Systematic characterization of biologically mediated exposure and risk is complicated by the abundance of microbial species, variation in dose-response between microorganisms, and the multiple factors affecting individual susceptibility across inhalation, fecal-oral, dermal, and other exposure routes (5).
Appreciable research has been conducted to understand pathogen exposure and provide risk management options among the general and consumer populations. Few guidance documents presenting semi-quantitative or quantitative frameworks, however, exist for the structured anticipation, recognition, evaluation, and control of workplace microbial hazards. Within occupational settings, bioaerosol exposure has been associated with a broad range of health effects including infectious and respiratory diseases, acute toxic effects, allergies, and cancer (1,3,6). The lack of a clear and transparent framework for providing a systematic approach to integrating laboratory, observational, clinical, and epidemiological evidence has been demonstrated during the COVID-19 pandemic when it became apparent that controlling exposure to bioaerosols (e.g., SARS-CoV-2) via the inhalation route was understood to be a temporally dynamic and environmentally complex process (7,8). Robust and comprehensive assessment and control strategies for pathogenic bioaerosols, especially in response to the COVID-19 pandemic, are therefore needed to reduce transmission risk at the workplace.
Occupational bioaerosol exposure is of particular concern across several industrial sectors, including waste recycling, food processing, agriculture, health care, travel (e.g., cruise ships), leisure (e.g., resorts and spas), manufacturing, and cannabis cultivation (1,6,9). Occupational exposures to bacteria and endotoxins, for example, are concerns among workers overseeing metalworking fluid tasks in manufacturing and fabrication workplaces (10). Wastewater treatment plant (WWTP) workers may be exposed to diverse aerosolized microorganisms, including SARS-CoV-2, in wastewater (7,11-13). Furthermore, the effect of SARS-CoV-2 transmission on business operations has generated substantial interest in occupational risk assessment tools for respiratory bioaerosols. Yet, industries have struggled to implement appropriate controls due to a lack of general knowledge, awareness, and training surrounding occupational microbial risk assessments (MRA) and control measures. These limitations likely stem from the lack of regulations and advisory standards specific to quantitative microbial risk assessments (QMRAs) for bioaerosols from U.S. regulatory agencies [e.g., U.S. Occupational Safety and Health Administration (OSHA)]; research agencies [e.g., National Institute for Occupational Safety & Health (NIOSH)]; or industry trade associations, as well as non-occupational U.S. and international agencies and groups [e.g., U.S. Environmental Protection Agency (EPA) or World Health Organization (WHO)] (14,15). As such, a need exists for addressing and expanding relevant regulations and standards that can aid health and safety (H&S) practitioners [e.g., industrial hygiene (IH) practitioners and occupational environmental health and safety (OEHS) practitioners] in their objective to better protect workers from microbial hazards.
The available health risk assessment paradigms describe a logical and stepwise approach for characterizing the potential for adverse health effects that may arise from exposure to the general population or occupational hazards (16). Risk assessment frameworks generally require synthesizing hazard, exposure, and dose-response information in order to characterize risk, and thus are similar regardless of hazard (e.g., microbial or chemical), route of exposure, or population of interest (e.g., consumer or occupational). These assessments may involve data that are quantitative, qualitative, or a hybrid of both types for assessing risk, and typically also evaluate the uncertainties associated with the process (16). Although considerable regulatory and industry experience in chemical and physical hazard risk assessment has been achieved over the last four decades, communicating standardized exposure assessment methods and dose-response information for microbial hazards has been lacking. The use of QMRA frameworks for microbial hazards has been increasingly proposed to encourage evidence integration (17). These approaches, however, have frequently been narrowly limited to application for one industry (e.g., WWTP or agriculture), pathogen, and/or route of exposure (15,17-19).
Using QMRAs can enhance and inform decision-making by providing numerical metrics, such as the risk of infection or disability-adjusted life years (DALYs), which can be further contextualized by quantitatively considering uncertainty and variability determinants. Historically, QMRAs have been applied to address microbial contamination in water and food to inform operational decisions, as well as prioritize control and risk mitigation efforts (20). These approaches, however, have been perceived as inaccessible or impractical because of their need for advanced technical knowledge and subject matter expertise. Additionally, applying a traditional QMRA approach requires data on specific pathogen occurrence, expected concentration, infectious dose, and survival characteristics, all of which are generally not readily available. These limitations and the technically intensive nature of QMRA frameworks have inhibited their widespread use in occupational risk management. To address this gap, a flexible semi-quantitative microbial risk assessment (sQMRA) framework was developed that is capable of application to less data- and model-dependent scenarios. Our goal is to help H&S practitioners take a systematic, data-driven, and flexible approach to risk-based decision-making in order to most effectively assess and manage workplace bioaerosol exposures. We present the following article in accordance with the Narrative Review reporting checklist (available at https://jphe.amegroups.com/article/view/10.21037/jphe-22-1/rc).
Methods
A search for peer-reviewed English-language literature and government documents published before October 19, 2021 was conducted to assess the current state of the science regarding QMRA in the context of occupational settings. Publications from political organizations and trade associations were excluded from the literature search. To identify published peer-reviewed literature, the following combination of search terms were used in PubMed, Google Scholar, and WorldWideScience: (“QMRA” OR “quantitative microbial risk assessment”) AND (“workplace” OR “worker” OR “occupational” OR “industrial hygiene” OR “occupational health”). The titles and abstracts of articles that met the inclusion criteria were initially reviewed for relevance, and publications that addressed the application of QMRA for bioaerosol characterization in occupational settings were reviewed in full. Additionally, relevant documents from government and authoritative international agencies related to QMRA in workplace settings were reviewed from the U.S. EPA, OSHA, California OSHA (Cal/OSHA), Centers for Disease Control and Prevention (CDC)-NIOSH, European Union (EU), WHO, and Food and Agriculture Organization of the United Nations (FAO). A summary of the literature search strategy is provided in Table 1.
Table 1
Items | Specification |
---|---|
Date of search | October 19, 2021 |
Databases and other sources searched | PubMed, Google Scholar, and WorldWideScience |
Search terms used | (“QMRA” OR “quantitative microbial risk assessment”) AND (“workplace” OR “worker” OR “occupational” OR “industrial hygiene” OR “occupational health”) |
Timeframe | Peer-reviewed articles and government documents published before October 19 2021 |
Inclusion and exclusion criteria | Articles that were unavailable in the English language and published after October 19, 2021, were excluded from this review. No additional limitations were placed on the date of publication or study design |
Selection process | The selection process included all authors of this manuscript during review of identified literature aligning with inclusion/exclusion criteria |
Any additional considerations | Government and authoritative international agency publications related to QMRA in workplace settings were reviewed from the U.S. EPA, OSHA, Cal/OSHA, CDC-NIOSH, EU, WHO, and FAO |
EPA, Environmental Protection Agency; OSHA, Occupational Safety and Health Administration; CDC, Centers for Disease Control and Prevention; NIOSH, National Institute for Occupational Safety & Health; EU, European Union; WHO, World Health Organization; FAO, Food and Agriculture Organization of the United Nations.
Discussion
The COVID-19 pandemic has illuminated appreciable gaps and limitations in microbial hazard awareness and response in the workplace. Furthermore, prominent training and resource gaps for microbial hazard assessment and management in the workplace have created the potential for inconsistent and ineffective risk management. Based on the inclusion criteria as described above, seven governmental and professional organization publications and 15 peer-reviewed publications were identified and evaluated for applicability and usability in the proposed sQMRA framework. These publications are discussed in more detail below.
Governmental and organization publications
Publicly available QMRA approaches and tools specific to the workplace were generally not available from governmental and professional organizations. This finding is consistent with the data gaps identified in the National Occupational Research Agenda, an agenda that highlights areas of critical research in the field of occupational H&S which is used by NIOSH to inform its strategic planning (21). Notably, developing models and the underlying data (e.g., dose-response relationships for pathogens) are an identified priority for reducing infectious disease incidence and transmission in the workplace (21). In addition, while the CDC has emphasized the importance of QMRA analyses for understanding SARS-CoV-2 fomite transmission, the agency has not communicated guidance for QMRA design or execution (22). No advisory or mandatory QMRA or MRA documentation was identified for bioaerosol or occupational applications among the relevant occupationally-focused U.S. governmental agencies.
Overall, workplace guidelines and applicable regulatory standards for microbial hazards are lacking at both the federal and state levels in the U.S. (14). The primary microbial-related standard at the federal level is OSHA’s bloodborne pathogen (BBP) standard, which provides methods for protecting workers from infectious pathogens in blood and other human bodily fluids (23). In the context of bioaerosol exposure in the workplace, however, the BBP standard does not explicitly cover respiratory secretions. In 2020, though, OSHA stated in its Guidance on Preparing Workplaces for COVID-19 that the BBP standard could offer a framework to help control some SARS-CoV-2 exposure sources (24). However, there was still a lack of guidance for comprehensive and robust frameworks from U.S. federal agencies, such as OSHA and NIOSH (14).
At the state level, Cal/OSHA established a more robust approach in 2001, publishing its guides “A Best Practices Approach for Reducing Bloodborne Pathogen Exposures” and an “Exposure Control Plan for Bloodborne Pathogens” (25,26). This overarching exposure control plan promoted using engineering controls and “more effective work practices” in healthcare settings (26). In 2009, Cal/OSHA published Section 5199 of the California Code of Regulations, regarding exposure controls for aerosol transmissible diseases in medical and clinical occupational settings as well as services that might otherwise be at increased bioaerosol contact risk, including laboratories, police services, homeless shelters, and correctional facilities (27). Specifically, Section 5199 requires that employers in such settings develop and implement infection control strategies in order to reduce transmission (27). Some examples include establishing vaccination and disease surveillance programs, screening and referring known and suspected cases of airborne infectious disease to healthcare facilities, implementing exposure controls such as physical barriers, personal protective equipment (PPE) (e.g., respiratory protection), and decontamination procedures, and evaluating air handling systems to ensure indoor air quality (27). Lastly, the standard dictates that employers should evaluate employee skills and knowledge and provide employee training to respond to existing and emerging microbial threats in order to help reduce transmission risk. The Cal/OSHA standard, however, is limited to a few industrial sectors and occupational tasks, and industry sectors outside of California may be unaware of its framework. A need exists at both the federal and state levels, then, to establish a regulatory framework and MRA tools to aid H&S practitioners across all industry sectors.
Among the non-occupationally focused regulatory agencies in the U.S., the EPA has established an MRA framework for waterborne pathogens. Specifically, the EPA published guidance establishing tools, methods, and approaches for conducting QMRAs that emphasized exposure to pathogens from water-related media (28). Applying a QMRA is a valuable approach for H&S practitioners, since it incorporates semi-quantitative and quantitative data into the MRA, allowing for quantitative risk determination. Additionally, the characteristics, benefits, and limitations of different static and dynamic modeling approaches are clearly described, and examples of generalized QMRA models are presented that are adaptable to any media or route of interest, with specific examples from the literature presented for waterborne pathogens only. While the EPA guidance provides comprehensive coverage of waterborne pathogens, it lacks similar guidance for bioaerosols and is not intended for conducting workplace QMRAs. Nonetheless, the QMRA guidance follows the essential steps of the National Research Council’s (NRC) 1983 risk assessment paradigm and thus is considered flexible and adaptable to other types of pathogen exposures (16,28).
The QMRA approaches proposed by non-occupational international agencies and groups similarly present limited guidance for assessing potential health risks associated with occupational bioaerosol exposures. These agencies and groups have released documentation and guidance for conducting QMRAs and MRAs targeted towards food and water [e.g., National Institute for Public Health and Environment of the Netherlands (RIVM), WHO, FAO/WHO, Health Canada (HC)]. These approaches contain a number of technical elements with high relevance and applicability to bioaerosols, as well as guidance for conducting general occupational QMRAs. The EU Directive 2000/54/EC, for example, prescribes minimum employer requirements for addressing biological agents in the workplace (29). The requirements emphasize the NIOSH Hierarchy of Controls, including eliminating exposures to potentially dangerous biological agents when possible, and ongoing assessment to account for any workplace changes affecting exposure potential (29,30). Determining the nature, degree, and duration of workers’ exposure is required; however, the directive does not require or suggest specific methods for conducting an MRA. In 2016, the WHO proposed QMRA guidance for fecal pathogens in drinking water, wastewater, and recreational settings (20). Procedures for bioaerosols, however, were not included (20). Fortunately, the available QMRA and MRA frameworks rely upon transparent and structured approaches consistent with the well-accepted health risk assessment paradigms, and thus can be readily adapted to develop a flexible, occupationally-focused bioaerosols framework.
Health-based agencies in countries outside the U.S. have also prepared MRA and QMRA documentation and guidance. Health Canada supports using QMRAs to characterize the microbiological content of water from the source to tap (31). The parameters of this model are limited to water, including considerations to account for specific disinfectant methods, such as coagulation and UV disinfection. Dose-response relationships, for example, account for common bacteria found in water derived from feeding trials and/or epidemiological data. In contrast, RIVM has published a more general tiered QMRA approach in order to accommodate changes in data availability and uncertainties to refine the quantitative assessment outcome across media, including food, water, and air (32). This tiered approach may be suitable for inhalation risk assessment after considering route-specific factors, such as indoor air ventilation characteristics, distance, and duration of exposure to the infected case.
Additional international public health agencies, including the WHO and the FAO, have published extensive MRA guidance for food. These approaches aim to support risk assessment (i.e., qualitative, semi-quantitative, and quantitative) of potential microbial hazards entering the food chain, excluding deliberate contamination (33). The WHO also proposed guidance to facilitate translating quantitative scientific data into water safety management practices in relation to risk associated with fecal pathogens, which may be applicable conceptually to other media (20). However, this MRA guidance does not focus on occupational exposures, or more specifically, bioaerosol exposures (20,33).
The general approaches to QMRAs for food and water are informative for biologically mediated respiratory diseases, such as COVID-19, when adapted to reflect the quantitative and conceptual considerations specific to the inhalation pathway. The methods used to collect and characterize exposure scenarios for potentially infectious bioaerosols, for example, may differ from those used in water and food, and, as such, meaningful differences may exist in risk determinants and uncertainty contributors. Information on the infectious dose, shedding concentration, exposure duration, and more (e.g., host susceptibility, secondary transmission, etc.) among cases for a target organism, for example, may be unknown. Uncertainties that detract from bioaerosol QMRA accuracy and validity may include assumptions about airborne concentration, associated dose, and exposure factors (e.g., distance and duration from a case). Variability also exists within biological systems, particularly for infectious dose and survival characteristics, which require additional data collection. Uncertainty and variability must therefore be accounted for in risk characterization and in risk management and evaluation. A thorough analysis of variability and uncertainty enables a better understanding of opportunities for refining assessments and facilitates a more adaptive assessment that can easily be updated as more information becomes available.
Current QMRA approaches may be difficult to implement and interpret without foundational infectious disease and prevention knowledge, and few guidelines have been developed to address the risk of infection in the workplace from bioaerosol exposure. Not surprisingly, adopting QMRA processes has been impaired by a lack of inter-disciplinary understanding regarding how the overarching risk assessment paradigm can be applied to microbial risk. The absence of such resources appears to, in part, reflect a lack of enforcement standards for microbes, particularly in the workplace (6). As has been demonstrated throughout the COVID-19 pandemic, supply shortages, lack of funding, and worker shortages can become commonplace during an infectious disease outbreak, further impairing the risk management process (15). Method development focused on effective approaches to conduct an occupational QMRA is therefore needed to mitigate exposure and occupational illness and disease risk.
Peer-reviewed publications
A total of 15 peer-reviewed publications encompassing QMRA approaches for various bacteria (including coliform), viruses (gastrointestinal and respiratory), fungi, and toxins were identified (11,13,18,34-45). The settings included WWTPs (seven studies), dental offices (one study), agriculture (two studies), biosolids and manure applications (one study), healthcare (one study), food processing (one study), academic (one study), and general population exposures (five studies), which are shown in Table 2.
Table 2
Industrial sector | Population(s) assessed | Microorganism(s) | Approach, key findings, and/or recommendations | Study |
---|---|---|---|---|
Agriculture | Dairy producers | E. coli O157:H7, C. perfringens, and Cryptosporidium spp. | Estimated both occupational and general population exposure via direct and indirect exposure scenarios using stochastic risk simulation methodology and reported potential for elevated risk near application sites | de Matos Nascimento et al., 2020, (36) |
Produce harvesters and packers (consumers) | Norovirus | Assessed whether interventions such as hand hygiene, worker furlough, and glove use reduced norovirus load, and found that combining various intervention methods (e.g., good hand washing compliance and efficacy) reduced risk to consumers and workers | Sobolik et al., 2021, (42) | |
Applied biosolids and manure | Occupations involving land-applied biosolids and general public | C. jejuni, E. coli O157:H7, L. monocytogenes, Salmonella spp., Adenovirus, Enterovirus, and Norovirus | Compared occupational and public risk of infection between different land application methods considering fomite, soil, crop, and aerosol exposures, and concluded that time and dilution were important risk mitigation factors | Brooks et al., 2011, (44) |
Dental office | Dental hygienists and patients | L. pneumophila (Legionella spp.) | Estimated effectiveness for interventions of N95 respirators for hygienists (95%), treatment to remove L. pneumophila (90% risk reduction per log reduction increase), and increased air exchange from 1.2 to 10 (85–88%) | Hamilton et al., 2021, (45) |
Engineered water systems | General public | Legionella spp. | Critically reviewed available Legionella QMRA approaches and concluded that the lack of standardized approaches could be addressed by the adoption of an enhanced conceptual model including aerosolization considerations such as the evolution of size distribution and the fate of bacteria contained within droplets | Hamilton and Haas, 2016, (39) |
Food Processing | Onion sorters | Penicillium, Aspergillus, Alternaria, Mucor, Fusarium species, and various mycotoxins | Illustrated the adoption of surrogate threshold limits intended for other, yet similar, scenarios to assess potential health risk of mycotoxins on onion skins, including consideration of European Commission regulations for unprocessed foodstuffs such as maize | Mayer et al., 2016, (40) |
Multiple sectors | Workers in healthcare settings and offices, WWTP, and solid waste landfills | Human adenovirus | Applied a QMRA methodology to assess HAdV in bioaerosol samples and reported that toilets had the highest probability of viral infection, followed by WWTPs and municipal solid waste landfills. However, the authors concluded that a more refined QMRA was necessary to inform risk management as only a sub-portion of the quantified adenovirus serotypes are associated with respiratory illness | Carducci et al., 2016, (18) |
Transportation | General public (bus transit) | SARS-CoV-2 | Presented a multi-factorial model considering activities and seating positions to assess relative risk and found a three-tiered risk assessment scheme was useful for relative risk classification of scenario | Ooi et al., 2021, (41) |
University Classrooms | Faculty and staff (students) | M. tuberculosis | Studied secondary attack rate in response to an outbreak and observed that increasing ventilation rates to reduce CO2 which resulted in a substantial decrease in the incidence of TB among contacts (after adjustments for contact investigation and latent TB treatment) | Du et al., 2020, (37) |
WRRF | WRRF workers | SARS-CoV-2 | Ranked risk of disease for SARS-CoV-2 exposures for four types of WRRF residuals based on the available literature and found existing recommended practices for handling raw sludge and Class A and B biosolids were sufficient to protect workers from SARS-CoV-2 | Brisolara et al., 2021, (34) |
WWTP | WWTP workers and nearby residents | Rotavirus, Adenovirus, hemorrhagic E. coli, Salmonella spp., Giardia spp., Cryptosporidium spp. | Demonstrated how the QMRA process can be used with the HACCP method to identify control points in the wastewater treatment process that can minimize exposure and concluded that the highest levels of risk were identified in the early treatment processes controllable by use of PPE and extended sludge treatment | Westrell et al., 2004, (43) |
S. aureus | Evaluated intervention effectiveness and reported that equipping workers and residents with PPE could decrease risk by at least an order of magnitude, and that QMRA is a useful educational “bridge” to better align perceived and estimated risks | Yan et al., 2021, (11) | ||
WWTP workers | SARS-CoV-2 | Utilized both wastewater and air samples of SARS-CoV-2 to determine infection risk from the QMRA model and recommended several risk management measures appropriate for bioaerosol producing processes including protective outerwear, gloves, goggles or face shield and mask, proper hand and personal hygiene, and consideration of administrative controls | Gholipour et al., 2021, (38) | |
Developed a QMRA approach to assess the risk of occupational exposure to SARS-CoV-2 among workers at WWTP for low-grade, moderate, or aggressive outbreaks, and concluded a low individual risk for low-grade and moderate outbreaks | Dada and Gyawali, 2021, (35) | |||
Applied QMRA to estimate risks for various exposure intensity scenarios using available surrogate data to develop recommendations for additional controls for scenarios exceeding a derived tolerable infection risk benchmark, including PPE and administrative controls | Zaneti et al., 2021, (13) |
QMRA, quantitative microbial risk assessment; HAdV, Human Adenovirus; WWTP, wastewater treatment plant; TB, tuberculosis; WRRF, water resource recovery facilities; HACCP, Hazard Analysis Critical Control Points; PPE, personal protective equipment.
Of the publications (Table 2), several themes and unique aspects regarding the application of QMRA and MRA approaches towards risk management decisions were identified. Across all studies, multiple sources of data were incorporated to inform each QMRA. Several studies evaluated control measure effectiveness during their assessment, including handwashing, air exchange, PPE, and administrative controls (11,13,38,42,43,45). Additionally, a few studies evaluated distance, duration, body orientation from the contamination source, and room ventilation characteristics (36,41,44). Lastly, several publications utilized data from microbial or physical surrogates (i.e., representative aerosols or gas), along with community outbreak data, to inform their QMRA (35,37,39). While no study directly addressed occupational QMRA frameworks for pathogenic bioaerosols, each provided valuable information pertaining to the approach and key considerations for conducting QMRAs. As such, the information collected from reviewing these studies has been deliberately incorporated into the proposed framework.
Proposed sQMRA framework and workflow
A sQMRA framework synthesizing the reviewed literature is proposed in Figure 1 for use by H&S practitioners during bioaerosol risk assessment and management in the workplace. This framework extends the NRC risk assessment paradigm by introducing considerations specific to workplace bioaerosols. Additionally, the proposed framework is complemented by a detailed step-by-step elaboration of the proposed process and information requirements (Figure 2). As described, the literature summarized in Table 2 was used to formulate the proposed sQMRA framework. Specifically, the data collected and utilized to determine risk in those studies were categorized. These categorized data inputs were used to define the variable inputs in the step-wise sQMRA framework proposed in Figures 1,2. The overall sQMRA framework is flexible as it allows for incorporating a combination of qualitative, semi-quantitative, and quantitative data, and provides examples of various inputs that can be utilized in the framework. Notably, the framework is designed to be used for scenario-specific evaluations that will have varying degrees of data availability, and is intended to be updated and refined as additional qualitative, semi-quantitative, and quantitative inputs become available. As such, as the amount of robust data increases through the incorporation of semi-quantitative and quantitative inputs the confidence of the sQMRA framework increases allowing for the reduction in uncertainty associated with the outputs.
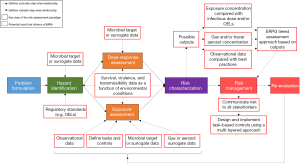
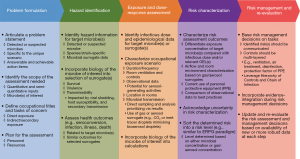
Problem formulation and hazard identification
The first step in assessing microbial hazards is to define the scope of the assessment and complete a problem formulation analysis. This step focuses the risk assessment on key questions pertaining to workplace microbial risk, culminating in a concise problem statement. This statement identifies the occupational tasks and job titles for which direct or indirect exposure and transmission may occur and ensures that resources address the key assessment questions. Problem formulation may be conducted in a proactive manner in anticipation of potential microbial hazards, or initiated during routine audits and compliance inspections, reported epidemiological factors (e.g., community cases and deaths) or outbreaks (e.g., COVID-19), or when a worker shows signs of infection (e.g., influenza).
Microbial hazard identification is performed early in the risk assessment within the context of the problem formulation and scope. In some settings, H&S practitioners may benefit from collaborating with epidemiologists, medical professionals, or infection preventionists when identifying the microbial species potentially associated with reported infections, signs, and symptoms. Information obtained on microbial hazards can be helpful for developing an initial understanding of typical characteristics, such as survival, virulence, or transmissibility. Microbial surrogates often represent similar biological attributes, including genetics, taxonomy, functional morphology, route of exposure, pathogenicity, and health effects, and can be helpful for addressing pathogen-specific data gaps and needs (46,47). This information can be incorporated into identifying and characterizing microbial hazards, and subsequently can inform the exposure and dose-response assessments. In cases where specific microbial species or serotypes are difficult to identify, or for which characterization results are not immediately available from the laboratory, hazard banding approaches informed using surrogate data can be considered as either a preliminary or final decision-making tool. Additionally, relevant regulatory or industry-specific standards should be consulted to evaluate whether the presence of microbial species would be considered out of compliance. Practitioners may also reference occupational exposure limits (OELs) established by regulatory or research agencies to understand the levels below which concentrations of the microbial species should be maintained. However, it should be noted that few OELs exist for microbial species with the exception of those developed for microbial byproducts (e.g., enzymes, toxins, and organic dusts). Therefore, multiple sources of evidence in addition to existing regulatory and industry-specific standards and OELs should be considered for the framework.
Exposure and dose-response assessment
Microbial-specific data from the hazard identification step can help inform the exposure assessment. Additional factors, such as exposure quantification and characterization, however, will need to be considered. Unfortunately, the lack of standardized sampling and analytical methods for bioaerosols necessitates incorporating multiple sources of evidence into the microbial risk assessment. Sources of evidence include data necessary to support the assessment described in the problem formation, such as duration and frequency of exposure, room ventilation and other exposure controls [e.g., ultraviolet-C (UVC) radiation or bipolar ionization], observational data, location in workplace and room where work is performed, and aerosol or gas surrogate data simulating bioaerosol transmission (e.g., CO2 or tracer droplets). Where available, these data inputs can be used in the sQMRA framework to refine the accuracy of exposure assessments for the microbial hazard(s) of interest for defined tasks in specific workplaces. As all desired data may not be immediately available, identifying the gaps in exposure assessment input, and seeking to fill those gaps will result in a more robust and comprehensive exposure assessment model that can be continually refined as additional data become available.
Dose-response assessments of chemical and physical hazards are well established compared to microbial hazards, which are complicated by lack of data, variability, and uncertainty. An infectious dose (i.e., the number of microbes of a particular species able to elicit an infection within a human), for example, is only known for a small fraction of pathogens, since extensive research is required to establish an infectious dose. This lack of data presents severe limitations for new and emerging pathogens (e.g., SARS-CoV-2). These assessments are further complicated by factors such as variability in case susceptibility, disease development, and severity. Additionally, some microorganisms also further complicate assessments by developing resistance to treatments or controls, allowing for environmental persistence or exponential growth. Based on the uncertainties and limitations currently surrounding pathogen infectious dose and dose-response assessments, exposure prevention efforts should prioritize other risk assessment steps. However, if dose-response data are available for a target or surrogate microorganism, then that information should be considered.
Risk characterization
After completion of the problem formulation, hazard identification, and exposure and dose-response assessments, the risk posed by the microbial hazard can be characterized. Historically, the risk from an occupational hazard for a defined task or scenario has been characterized by the likelihood of exposure to the hazard based on the exposure assessment analysis and the severity of the health outcome identified during the hazard identification step. This risk characterization step can result in varying outcomes, including a comparison of exposure to established OELs, infectious dose, gas and/or tracer aerosol concentration illustrating airflow, or observational data of the occupational task compared with best practices. Furthermore, these outcomes can be refined to characterize the risk more robustly and conservatively by incorporating them into a tiered assessment approach in order to inform risk management decisions, similar to those established by the American Industrial Hygiene Associations (AIHA) Emergency Response Planning Committee (ERP Committee). The ERP Committee established Emergency Response Planning Guidelines (ERPGs), which are air concentration guidelines for assessing accident prevention and emergency response plan adequacy (48).
By leveraging an approach similar to the ERPG, a risk level can be determined for occupational exposure to a microbial hazard by incorporating data pertaining to the target microbe, a microbial surrogate, or gas/aerosol surrogate. Notably, this approach has already been proposed by Ooi et al. (41), in which a three-tiered risk assessment scheme for either computational fluid dynamic (CFD) simulations or microbial and gas/aerosol surrogate data was proposed. This approach allows more flexibility for determining risk levels along with subsequent risk management decisions in order to reduce exposure to the target microbe. Because of uncertainty surrounding various aspects of the sQMRA (e.g., dose-response), however, risk levels should be developed in a conservative manner in order to account for the framework’s associated uncertainties and variability.
Risk management and re-evaluation
Once a risk level has been estimated, risk management decisions specific to the job task of interest should be considered, especially if there are applicable regulatory or industry-specific standards. These considerations should include communicating identified risks to workers and relevant stakeholders (e.g., contractors or visitors) and implementing control measures to reduce exposure. Additionally, selecting workplace controls can be guided by a hybrid approach of the NIOSH Hierarchy of Controls and Chain of Infection paradigms proposed by Zisook et al. (15). By leveraging both paradigms simultaneously, controls can be multilayered (e.g., ventilation, air and surface treatment, and PPE) and flexible. Also, implementing controls that are likely to reduce multiple bioaerosol hazards will allow for a protective approach for managing multiple bioaerosol constituents or unknowns. Approaches to exposure control should also remain flexible in order to accommodate new and emerging evidence, regulation changes, and modifications for best practices. Additionally, this flexibility allows for a process that can be re-evaluated and updated as scientific understanding advances and data becomes available to address sQMRA model assumptions.
Case scenario
A hypothetical case scenario addressing the possible risk of exposure and subsequent SARS-CoV-2 transmission during the performance of a generic occupational task is presented in Figure 3 to illustrate our proposed sQMRA approach. In this scenario, four workers on the same shift and involved with Task A have been diagnosed with COVID-19 after developing symptoms consistent with SARS-CoV-2 infection (e.g., coughing, fatigue, shortness of breath). A risk of occupational exposure and subsequent transmission of SARS-CoV-2 to workers therefore was hypothesized to be associated with Task A. The identified hazard (i.e., contact of SARS-CoV-2 aerosol with eyes or respiratory tract) may lead to COVID-19-related disease (including respiratory distress) based on emerging reports of serious health outcomes from the local public health department. Consensus dose-response data and validated exposure assessment methods, however, are lacking. Thus, a literature review and weight of evidence evaluation of microbial and aerosol surrogate data were used in conjunction with a transparent assessment of uncertainties inherent in “read across” assessments involving surrogate data in order to inform the Task A risk characterization.
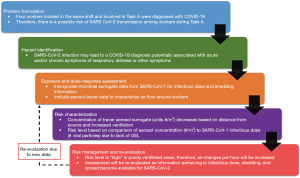
Based on the Task A hazard identification and exposure assessment, risk is characterized by determining the concentration of intentionally released tracer aerosol (i.e., physical surrogate) in the workplace across distance and ventilation airflow. The tracer aerosol is intended to mimic the respiratory aerosol airflow in the location where Task A is performed in the workplace. Furthermore, due to the lack of an OEL, a quantitative risk level is determined by comparing the tracer aerosol concentration to the reported SARS-CoV-1 (i.e., microbial surrogate) infectious dose [i.e., risk level = aerosol concentration (#/m3)/SARS-CoV-1 infectious dose (# viral particles)]. The hypothetical risk level is determined to be “high” in poorly ventilated areas when the aerosol tracer concentration is higher than the reported infectious dose for SARS-CoV-1. This hypothetical “high” risk level criterion is based on the current risk policy developed for the specific workplace. As part of the subsequent engineering evaluation, industrial ventilation guidelines are consulted, and a subcontractor is asked to perform a focused CFD simulation assessing several design options. Based on the CFD simulation assessment, a risk management decision is therefore made to increase ventilation in areas in which Task A is performed. After increasing ventilation, the control needs to be re-evaluated for effectiveness. Increasing ventilation in an area, for example, could also lead to higher source contamination based on the locations of the source, the exposed employee, the supplied air path, and the exhaust vent. Throughout the pandemic, implementing barriers as a control resulted in obstructing adequate ventilation and demonstrated the importance of control re-evaluation. Additionally, the hypothetical risk level will be re-evaluated as new or more robust data regarding infectious dose become available.
The proposed sQMRA framework and workflow described above are designed to be applied to any workplace and task. The framework therefore will need to be optimized for the industry, workplace, and task in which it is being applied by H&S practitioners on a case-by-case basis. The framework is semi-quantitative, as it allows for the integration of multiple forms of data in which risk characterization can still be determined based on the risk level. Additionally, the framework has been designed to complement validated approaches (e.g., NRC) and be less data-intensive and technical, such as those proposed in scientific literature. By applying a systematic and semi-quantitative approach, the framework allows scientific evidence to be evaluated and continually integrated as it becomes available. The inherent flexibility of the proposed approach to sQMRAs is vital during emergency situations and when attempting to control exposure to highly pathogenic microbes. During the COVID-19 pandemic, for example, the sQMRA’s flexibility, evaluation, and adaptation for SARS-CoV-2 was essential, as the scientific evidence continued to evolve, and occasionally resulted in conflicting recommendations for exposure control measures.
An impactful and effective QMRA framework should include the scientific advances being developed as part of the emerging NIOSH Total Worker Health® (TWH) paradigm. Under the leadership of NIOSH, TWH focuses on integrating policies, programs, and practices that provide synergistic benefits to employer, worker, and community well-being (49). Zisook et al. (15) illustrated how the Hierarchy of Controls adopted in response to the COVID-19 pandemic can be applied to the TWH concepts of elimination, substitution, redesign, education, and encouragement (50). Under a TWH-informed approach, a more sophisticated QMRA is possible by considering that transmission of pathogens is not isolated to the workplace, and can occur in multiple directions, either to or from the workplace (i.e., multi-directional). A multi-directional TWH approach for infectious bioaerosols enables robust and holistic controls and helps encourage personal decision making and corporate risk management with a shift in focus from individual harm reduction to a supportive, shared workplace team and community experience of well-being.
The proposed framework is a tool intended to help protect workers from microbial hazards by assessing the risks in order to inform appropriate risk management response measures in a systematic and data-driven manner. More information, however, is continually needed to better characterize microbial hazard, exposure, and risk determination.
Limitations
The proposed sQMRA framework is a general framework designed to be adapted to occupational settings in which bioaerosol exposure may occur. As such, when applied, practitioners may optimize the framework to suit the specific microbe and conditions of the workplace, given available data. As currently outlined, however, this framework does not provide specific guidance as to an acceptable risk level. Instead, an acceptable risk level should be determined by the practitioner for the specific scenario being evaluated. Furthermore, the framework does not detail available dose-response models for evaluating infectious dose, which are available to practitioners should they require more specific data for the microbe of interest in their assessment. A lack of suitable target organism data that addresses determinants such as survival, virulence, and transmissibility represent an important limitation of the proposed approach in some scenarios, especially for complex populations of microorganisms found among some bioaerosols. Surrogate microbial data, however, provides a reasonable basis for quantifying potential risk until additional information becomes available. In situations where several pathogenic microorganisms are identified among bioaerosols in the workplace, a practitioner may utilize this framework by focusing on the microorganism considered the most pathogenic to inform overall risk management decisions. Additionally, if the need arises, the sQMRA framework may be used multiple times for multiple organisms contained within the same bioaerosol profile to determine overlapping or conflicting risk management options based on the risk assessment. Irrespective of the data availability, the usefulness of the framework requires an assessment and transparent characterization of uncertainty and variability.
Conclusions
Exposure to pathogenic bioaerosols in the workplace can occur across industrial sectors from either community transmission or specific operations and tasks unique to a particular industry. In fact, outbreaks in the workplace have been regularly reported involving norovirus, influenza, Legionella, endotoxins, and several other bacteria and fungi. Understanding the exposure and transmission dynamics of these pathogens has been emphasized following the COVID-19 pandemic, resulting in a need for more standardization in the workplace, and accessibility of resources for H&S practitioners. This need also extends to QMRAs. Based on the identified literature, however, few QMRA resources for occupational bioaerosol exposure exists for use by H&S practitioners. As such, the authors have proposed a flexible and data-driven sQMRA framework that can be optimized to each unique task, workplace, or industry. This framework represents the first step towards providing more resources and tools that are easily implementable and adaptable to any occupational environment.
Acknowledgments
The authors would like to thank Carrie Kahn for her review and assistance in preparing this manuscript.
Funding: None.
Footnote
Provenance and Peer Review: This article was commissioned by the Guest Editors (Kenneth A. Mundt and Justine Parker) for the series “Managing COVID-19 Risks in the Workplace” published in Journal of Public Health and Emergency. The article has undergone external peer review.
Reporting Checklist: The authors have completed the Narrative Review reporting checklist. Available at https://jphe.amegroups.com/article/view/10.21037/jphe-22-1/rc
Conflicts of Interest: All authors have completed the ICMJE uniform disclosure form (available at https://jphe.amegroups.com/article/view/10.21037/jphe-22-1/coif). The series “Managing COVID-19 Risks in the Workplace” was commissioned by the editorial office without any funding or sponsorship. CB, RZ, ANB, CH, DL, RKB, and KMU are currently employed by Cardno ChemRisk now Stantec, and CM was formerly employed by Cardno ChemRisk now Stantec. CB, RZ, and KMU have served as contributors to the AIHA Back to Work Safely Task Force by drafting industry-specific guidance for businesses and consumers to safely reopen after emerging from COVID-19 shelter-in-place/quarantine. KKM is a System-Wide Industrial Hygienist at Cone Health, but the views expressed in this research do not reflect views of Cone Health. The authors have no other conflicts of interest to declare.
Ethical Statement: The authors are accountable for all aspects of the work in ensuring that questions related to the accuracy or integrity of any part of the work are appropriately investigated and resolved.
Open Access Statement: This is an Open Access article distributed in accordance with the Creative Commons Attribution-NonCommercial-NoDerivs 4.0 International License (CC BY-NC-ND 4.0), which permits the non-commercial replication and distribution of the article with the strict proviso that no changes or edits are made and the original work is properly cited (including links to both the formal publication through the relevant DOI and the license). See: https://creativecommons.org/licenses/by-nc-nd/4.0/.
References
- Douwes J, Thorne P, Pearce N, et al. Bioaerosol health effects and exposure assessment: progress and prospects. Ann Occup Hyg 2003;47:187-200. [PubMed]
- Kim KH, Kabir E, Jahan SA. Airborne bioaerosols and their impact on human health. J Environ Sci (China) 2018;67:23-35. [Crossref] [PubMed]
- Lindsley WG, Green BJ, Blachere FM, et al. Sampling and characterization of bioaerosols. National Institute for Occupational Safety and Health, 2017.
- Georgakopoulos DG, Després V, Fröhlich-Nowoisky J, et al. Microbiology and atmospheric processes: biological, physical and chemical characterization of aerosol particles. Biogeosciences 2009;6:721-37. [Crossref]
- Brouwer AF, Weir MH, Eisenberg MC, et al. Dose-response relationships for environmentally mediated infectious disease transmission models. PLoS Comput Biol 2017;13:e1005481. [Crossref] [PubMed]
- (ACGIH) American Conference of Governmental Industrial Hygienists. Bioaerosols: assessment and control. ACGIH Bioaerosols Committee, 1999.
- Kitajima M, Ahmed W, Bibby K, et al. SARS-CoV-2 in wastewater: State of the knowledge and research needs. Sci Total Environ 2020;739:139076. [Crossref] [PubMed]
- Yao M, Zhang L, Ma J, et al. On airborne transmission and control of SARS-Cov-2. Sci Total Environ 2020;731:139178. [Crossref] [PubMed]
- Couch JR, Grimes GR, Wiegand DM, et al. Potential occupational and respiratory hazards in a Minnesota cannabis cultivation and processing facility. Am J Ind Med 2019;62:874-82. [Crossref] [PubMed]
- Marchand G, Lavoie J, Racine L, et al. Evaluation of bacterial contamination and control methods in soluble metalworking fluids. J Occup Environ Hyg 2010;7:358-66. [Crossref] [PubMed]
- Yan C, Leng YL, Wu JT. Quantitative microbial risk assessment for occupational health of temporary entrants and staffs equipped with various grade PPE and exposed to microbial bioaerosols in two WWTPs. Int Arch Occup Environ Health 2021;94:1327-43. [Crossref] [PubMed]
- Yan C, Wang RN, Zhao XY. Emission characteristics of bioaerosol and quantitative microbiological risk assessment for equipping individuals with various personal protective equipment in a WWTP. Chemosphere 2021;265:129117. [Crossref] [PubMed]
- Zaneti RN, Girardi V, Spilki FR, et al. Quantitative microbial risk assessment of SARS-CoV-2 for workers in wastewater treatment plants. Sci Total Environ 2021;754:142163. [Crossref] [PubMed]
- Boles C, Parker J, Hallett L, et al. Current understanding and future directions for an occupational infectious disease standard. Toxicol Ind Health 2020;36:703-10. [Crossref] [PubMed]
- Zisook RE, Monnot A, Parker J, et al. Assessing and managing the risks of COVID-19 in the workplace: Applying industrial hygiene (IH)/occupational and environmental health and safety (OEHS) frameworks. Toxicol Ind Health 2020;36:607-18. [Crossref] [PubMed]
- Council NR. Risk assessment in the federal government: managing the process. 1983.
- Petterson SR. Application of a QMRA Framework to Inform Selection of Drinking Water Interventions in the Developing Context. Risk Anal 2016;36:203-14. [Crossref] [PubMed]
- Carducci A, Donzelli G, Cioni L, et al. Quantitative Microbial Risk Assessment in Occupational Settings Applied to the Airborne Human Adenovirus Infection. Int J Environ Res Public Health 2016;13:733. [Crossref] [PubMed]
- Madera-García V, Mraz AL, López-Gálvez N, et al. Legionella pneumophila as a Health Hazard to Miners: A Pilot Study of Water Quality and QMRA. Water (Basel) 2019;11:1528. [Crossref] [PubMed]
- World Health Organization (WHO). Quantitative microbial risk assessment: Application for water safety management. Geneva, Switzerland; 2016. Available online: https://apps.who.int/iris/handle/10665/246195
- Centers for Disease Control and Prevention (CDC). National Occupational Research Agenda. Immune, Infectious and Dermal Disease Prevention (IID). 2019.
- Centers for Disease Control and Prevention (CDC). SARS-CoV-2 and surface (fomite) transmission for indoor community environments. COVID-19 Science Briefs, 2021.
- Occupational Safety and Health Administration. Occupational Safety and Health Standards. Bloodborne pathogens. 1910.1030; 1991.
- Occupational Safety and Health Administration. Guidance on preparing workplaces for COVID-19. 2020. Available online: https://www.osha.gov/sites/default/files/publications/OSHA3990.pdf
- California Division of Occupational Safety and Health. A Best Practices Approach for Reducing Bloodborne Pathogens Exposure. 2001. Available online: https://www.dir.ca.gov/dosh/dosh_publications/bbpbest1.pdf
- California Division of Occupational Safety and Health. Exposure Control Plan for Bloodborne Pathogens. 2001.
- California Division of Occupational Safety and Health. Standard 5199 - Aerosol Transmissable Diseases. 2009. Available online: https://www.dir.ca.gov/dosh/dosh_publications/expplan2.pdf
- Environmental Protection Agency. Microbiological risk assessment (MRA) tools, methods, and approaches for water media. 2014. Available online: https://www.epa.gov/sites/default/files/2015-11/documents/microbial-risk-assessment-mra-tools-methods-and-approaches-for-water-media.pdf
- European Parliament. Directive 2000/54/EC of the European Parliament and of the Council of 18 September 2000 on the protection of workers from risks related to exposure to biological agents at work (seventh individual directive within the meaning of Article 16 (1) of Directive 89/391/EEC). Official Journal of the European Communities 2000;5:0021-45.
- National Institute for Occupational Safety and Health. Hierarchy of Controls. 2015. Available online: https://www.cdc.gov/niosh/topics/hierarchy/default.html
- Health Canada. Guidance on the Use of Quantitative Microbial Risk Assessment in Drinking Water. Water and Air Quality Bureau HEaCSB, 2019. Available online: https://www.canada.ca/en/health-canada/programs/consultations-guidance-quantitative-microbial-risk-assessment-drinking-water/document.html
- Teunis P, Schijven J. Generic guidance to quantitative microbial risk assessment for food and water. 2019.
- (WHO) World Health Organization. Microbiological Risk Assessment–Guidance for food. Food & Agriculture Organization (FAO); 2021. Available online: https://www.who.int/publications/i/item/9789240024892
- Brisolara KF, Maal-Bared R, Sobsey MD, et al. Assessing and managing SARS-CoV-2 occupational health risk to workers handling residuals and biosolids. Sci Total Environ 2021;774:145732. [Crossref] [PubMed]
- Dada AC, Gyawali P. Quantitative microbial risk assessment (QMRA) of occupational exposure to SARS-CoV-2 in wastewater treatment plants. Sci Total Environ 2021;763:142989. [Crossref] [PubMed]
- de Matos Nascimento A, de Paula VR, Dias EHO, et al. Quantitative microbial risk assessment of occupational and public risks associated with bioaerosols generated during the application of dairy cattle wastewater as biofertilizer. Sci Total Environ 2020;745:140711. [Crossref] [PubMed]
- Du CR, Wang SC, Yu MC, et al. Effect of ventilation improvement during a tuberculosis outbreak in underventilated university buildings. Indoor Air 2020;30:422-32. [Crossref] [PubMed]
- Gholipour S, Mohammadi F, Nikaeen M, et al. COVID-19 infection risk from exposure to aerosols of wastewater treatment plants. Chemosphere 2021;273:129701. [Crossref] [PubMed]
- Hamilton K, Haas C. Critical review of mathematical approaches for quantitative microbial risk assessment (QMRA) of Legionella in engineered water systems: research gaps and a new framework. Environ Sci Water Res Technol 2016;2:599-613. [Crossref]
- Mayer S, Twarużek M, Błajet-Kosicka A, Grajewski J. Occupational exposure to mould and microbial metabolites during onion sorting--insights into an overlooked workplace. Environ Monit Assess 2016;188:154. [Crossref] [PubMed]
- Ooi CC, Suwardi A, Ou Yang ZL, et al. Risk assessment of airborne COVID-19 exposure in social settings. Phys Fluids (1994) 2021;33:087118.
- Sobolik JS, Newman KL, Jaykus LA, et al. Norovirus transmission mitigation strategies during simulated produce harvest and packing. Int J Food Microbiol 2021;357:109365. [Crossref] [PubMed]
- Westrell T, Schönning C, Stenström TA, et al. QMRA (quantitative microbial risk assessment) and HACCP (hazard analysis and critical control points) for management of pathogens in wastewater and sewage sludge treatment and reuse. Water Sci Technol 2004;50:23-30. [Crossref] [PubMed]
- Brooks JP, McLaughlin MR, Gerba CP, et al. Land application of manure and Class B biosolids: an occupational and public quantitative microbial risk assessment. J Environ Qual 2012;41:2009-23. [Crossref] [PubMed]
- Hamilton KA, Kuppravalli A, Heida A, et al. Legionnaires' disease in dental offices: Quantifying aerosol risks to dental workers and patients. J Occup Environ Hyg 2021;18:378-93. [Crossref] [PubMed]
- Hu M, Gurtler JB. Selection of Surrogate Bacteria for Use in Food Safety Challenge Studies: A Review. J Food Prot 2017;80:1506-36. [Crossref] [PubMed]
- Sinclair RG, Rose JB, Hashsham SA, et al. Criteria for selection of surrogates used to study the fate and control of pathogens in the environment. Appl Environ Microbiol 2012;78:1969-77. [Crossref] [PubMed]
- (AIHA) American Industrial Hygiene Association. Emergency Response Planning Guidelines. American Industrial Hyigene Association (AIHA). 2021. Available online: https://www.aiha.org/get-involved/aiha-guideline-foundation/erpgs.
- (NIOSH) National Institute for Occupational Safety and Health. Fundamentals of Total Worker Health Approaches: Essential Elements for Advancing Worker Safety, Health, and Well-Being. 2016. Available online: https://www.cdc.gov/niosh/docs/2017-112/pdfs/2017_112.pdf
- (NIOSH) National Institute for Occupational Safety and Health. Hierarchy of Controls Applied to NIOSH Total Worker Health. 2018. Available online: https://www.cdc.gov/niosh/twh/guidelines.html#:~:text=use%20this%20hierarchy-,The%20%E2%80%9CHierarchy%20of%20Controls%20Applied%20to%20NIOSH%20Total%20Worker%20Health,otherwise%20negatively%20impact%20well%2Dbeing
Cite this article as: Boles C, Zisook R, Buerger AN, Hamaji C, Mathis C, Lauer D, Brewster RK, Meachum KK, Nonnenmann M, Unice KM. Semi-quantitative microbial risk assessment: a narrative review and proposed framework for health and safety practitioners. J Public Health Emerg 2022;6:34.