Literature review of the relative importance of household, community and social, and workplace settings on the probability of COVID-19 infection
Introduction
Coronavirus disease 2019 (COVID-19) is a highly infectious respiratory disease caused by severe acute respiratory syndrome coronavirus 2 (SARS-CoV-2), which emerged in Wuhan province, China, in late 2019 (1). SARS-CoV-2 is largely spread via direct, indirect, or close contact with infected individuals’ respiratory secretions, respiratory droplets, or fine aerosol particles from coughs, sneezes, or talking/singing (2). The transmissibility of SARS-CoV-2 may fluctuate due to changes in viral kinetics, including the rate of viral replication, peak viral load, and viral clearance. Viral kinetics also may change with vaccination status and genetic variation in SARS-CoV-2, including the emergence of new variants (3). Indeed, Delta and Omicron variants have been associated with dramatic increases in transmissibility and infectivity relative to earlier variants of SARS-CoV-2, and the latter also appears to cause less severe symptoms resulting in lower case hospitalization rates (4).
In any potential exposure scenario, the likelihood of infection by SARS-CoV-2 is driven by the virus’ inherent transmissibility coupled with various exposure parameters, as well as individual behavioral and environmental risk factors. Fundamentally, however, given that COVID-19 is a community-spread disease, the baseline risk of infection is closely linked to community rates of infection at a given point in time (quantified using the basic reproduction rate [R naught (R0)], which reflects the average number of other people an infected individual will infect) (5). Because each individual has their own (likely overlapping) network of community contacts—and each individual in the network will have their own network of community contacts—the number of potential sources of infection increases exponentially with increasing contacts. Complicating this further are the time-dependent aspects of transmission, as individuals with COVID-19 are infective for a specific time period. Furthermore, some proportion of infected individuals will have no or only mild symptoms (that they may not associate with COVID-19) but nevertheless be infective for several days following their becoming infected.
Behavioral factors affecting risk may include, but are not limited to, modes and pattern of travel, frequency and duration of time spent outside the home, frequency, duration and characteristics of social interactions, use of personal protective equipment (e.g., masks, respirators), and personal hygiene (e.g., handwashing, hand sanitizer use). Similarly, environmental factors, such as room size, room ventilation rate and airflow characteristics, as well as characteristics of the exposure, including duration of contact with individuals infected with SARS-CoV-2 (with or without symptoms), number of cases to which one is exposed, and physical distance from COVID-19 case(s), affect the probability of transmission.
Throughout the course of the COVID-19 pandemic, now in its third year, outbreaks have occurred in virtually all settings, including household, community, social, and workplace environments—even in the most remote regions of the world (6). Our objective was to review the current evidence regarding the primary risk factors, settings and scenarios that are associated with SARS-CoV-2 transmission and COVID-19 risk that also may be informative for or considered in analyses of the relative probability of developing COVID-19 for specific exposure conditions. We present the following article in accordance with the Narrative Review reporting checklist (available at https://jphe.amegroups.com/article/view/10.21037/jphe-22-12/rc).
Methods
A literature review was conducted to identify articles assessing the potential risk of SARS-CoV-2 transmission among individuals in various household and non-household settings. Articles were included if they were in the English language, a human study, peer-reviewed, and reported a quantitative outcome. Articles were excluded if they were in vivo or in vitro laboratory studies, case reports, commentaries, had a model-based outcome, or only assessed transmission risk in healthcare workers in a clinical occupational setting.
We performed a literature search in December 2021 using the PubMed online database using the following search criteria: (covid-19) AND LitCTRANSMISSION[filter] AND ((factors) OR (factor) OR (risk) OR (likelihood) OR (increase) OR (probability) OR (exposure)). Filters for “English” and “Human” studies were applied to this search, and no date limits were applied. We also used a special filter specific to COVID-19 in PubMed called “LitCTransmission,” which is shorthand for the following search terms:
“(“COVID-19” OR “COVID-19”[MeSH Terms] OR “COVID-19 Vaccines” OR “COVID-19 Vaccines”[MeSH Terms] OR “COVID-19 serotherapy” OR “COVID-19 serotherapy”[Supplementary Concept] OR “COVID-19 Nucleic Acid Testing” OR “covid-19 nucleic acid testing”[MeSH Terms] OR “COVID-19 Serological Testing” OR “covid-19 serological testing”[MeSH Terms] OR “COVID-19 Testing” OR “covid-19 testing”[MeSH Terms] OR “SARS-CoV-2” OR “sars-cov-2”[MeSH Terms] OR “Severe Acute Respiratory Syndrome Coronavirus 2” OR “NCOV” OR “2019 NCOV” OR ((“coronavirus”[MeSH Terms] OR “coronavirus” OR “COV”) AND 2019/11/01[PDAT] : 3000/12/31[PDAT])) AND (“transmission”[Text Word] OR “transmission”[MeSH Subheading] OR “replication”[Text Word] OR “disease transmission, infectious”[MeSH Terms])”.
Additional articles were identified from cross-referencing relevant article references, weekly PubMed email listserv communications, and non-systematic Google Scholar searches. From these searches, articles were assessed for eligibility in an initial title and abstract review, followed by a full text review. If studies were found eligible after the two-step review process, the data regarding risk and attack rates (AR) for different settings where SARS-CoV-2 was transmitted were extracted and reported in the supplementary table at https://cdn.amegroups.cn/static/public/10.21037jphe-22-12-1.xlsx.
Results
The PubMed search yielded 9,230 results. A total of 9,147 studies were excluded after reviewing the title and abstract, and another 51 were excluded after full-text screening, largely due to a failure to include quantitative data. An additional 13 potentially relevant studies were identified through other means, primarily mining of review article references and weekly PubMed screenings checked continually throughout this research. A total of 33 studies met inclusion criteria for full-text review and data abstraction. Most studies reported either secondary attack rates (SARs) of COVID-19 infection or odds/risk ratios associated with household, community/social, and workplace settings, risk factors, and/or behaviors. The specific types of settings and underlying risk factors associated with COVID-19 infection risk in the identified literature in each of these settings are depicted in Figure 1.
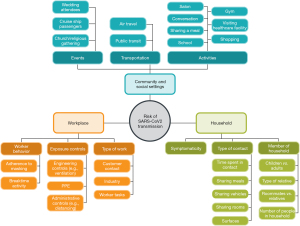
Household settings
Across the studies reviewed, SARs for household settings ranged from 9.7% to 69% (7-19). Most studies evaluated the relative importance of several environments, including the household, healthcare settings, and the workplace; in some cases, public transportation/travel, dining, mass gatherings, and other settings. In all studies evaluating household versus other transmission settings, the AR or risk of COVID-19 infection was highest in the household setting (20,21).
The characteristics of the household interactions with an index case or cases (e.g., contact time, type of interaction, number of cases in the household) may also affect likelihood of infection. In a prospective cohort study of cases in Hubei Province, China, at the start of the pandemic, Xin et al. (18) reported that risk of COVID-19 among household members increased with the number of effective contact days (i.e., 3–11 or ≥11 days, relative to 1–2 days). However, in another study conducted in early 2020 in Zhuhai, China, Wu et al. (17) reported that there was no difference in the likelihood of infection in those with <72 versus ≥72 hours of total time at home with the index case; however, the maximum total time with the case was not reported.
In a retrospective cohort study of close contacts of cases in Singapore (January-April, 2020) sharing a bedroom (versus not sharing) and speaking to a case ≥30 minutes (relative to <30 minutes) were associated with an increased risk of infection in households (OR =5.38, 95% CI: 1.82–15.84; P=0.0023 for bedroom sharing; OR =7.86, 95% CI: 3.86–16.02, P<0.0001 for ≥30 minutes of speaking) (22). Sharing a toilet, sharing meals, indirect contact (touching surfaces/objects touched by cases), and sharing of a vehicle were not associated with significantly increased risk of infection in multivariable analyses (22). In contrast, Wu et al. (17) reported statistically significantly increased odds of COVID-19 infection with shared vehicles, shared meals, and a shared living room (ORs ranged from 2.54 to 3.49). Ng et al. (22) reported that contact with more than one household COVID-19 case was statistically significantly associated with increased risk of infection, relative to contact with only a single case. Wu et al. (17) reported no statistically significant associations between number of positive cases within the household and secondary infections.
Two studies evaluated the effect of overcrowding on risk of SARS-CoV-2 infection; these studies generally demonstrated that crowded conditions increase infection risk. For example, Ghosh et al. (23) conducted a study of 39,923 COVID-19 cases presenting to emergency rooms in New York City in March of 2020, and investigated transmission associated with household over-crowdedness (as defined by more than one occupant per room) and multigenerational households. Household over-crowdedness was associated with a modest, but statistically significant, increase in risk of COVID-19 relative to uncrowded households, with slight increases in magnitude of risk with increased overcrowding. Similar findings were reported for multi-generation households. Burke et al. (7) reported that presumed transmission (based on symptoms where test results not available) was more common in households with ≥5 members relative to those with fewer than 5 members (78% versus 39%, P=0.005). In a study of farmworkers, there was a positive association between crowded housing and infection, although these results were not statistically significant (21).
Several studies evaluated whether the specific infected family member or roommate (e.g., spouse versus a child, relative versus a non-relative) affected household transmission (7,17,21,22). Burke et al. (7) reported a SAR of 54% in households; interestingly, the SARS-CoV-2 transmission rate from children to their parents was statistically significantly higher than from an adult to their spouse. However, in Ng et al. (22), spouses/partners of COVID-19 cases were statistically significantly more likely to be infected with COVID-19 relative to non-family member contacts in univariable, but not multivariable analyses. Further, Wu et al. (17) reported statistically significantly increased risk of transmission in spouses of index cases, but no significant increase in other first-degree relatives, compared to more distant relatives. Chaw et al. (8) indicated that close contact with infected spouses had the highest risk of infection with COVID-19, but the risk was also statistically significantly increased for contact with children. The risk of infection from close contact with siblings, parents, housekeepers, or relatives, such as grandparents and grandchildren, was not statistically significantly increased. Similarly, Jashaninejad et al. (24) reported that, among household close contacts of index cases, spouses and parents/grandparents were most likely to be infected, relative to the referent group of grandchildren (children and sister/brothers showed no significantly increased risk relative to grandchildren). Mora et al. (21) found that the relative risk of COVID-19 infection was slightly higher for farmworkers living with roommates that were not relatives versus family members (RR =1.40, 95% CI: 1.19–1.64) and for workers who lived with children ≤5 years old versus those that did not (RR =1.40, 95% CI: 1.11–1.76).
Finally, a limited number of studies have evaluated the effect of symptoms and severity of disease on transmission. It is theorized that disease severity may be a surrogate for symptomaticity and duration of illness. Further, increased severity may be associated with increased aerosol generation or higher viral load in symptomatic and severe cases, relative to asymptomatic and mild cases, respectively. In a cross-sectional study, Kahlert et al. (12) evaluated household transmission among hospital workers in several European countries, reporting a higher risk of COVID-19 in secondary cases if the close contact was symptomatic with respiratory symptoms relative to asymptomatic (OR =3.02, 95% CI: 2.12–4.32, P<0.001). In contrast, Hu et al. (19) found no statistically significant difference in transmissibility between symptomatic (fever and coughing were most prevalent) and asymptomatic/presymptomatic individuals, although there were fewer pre-symptomatic cases identified (n=43). Similarly, Wu et al. (17) found no association between fever and infection risk, but there was a borderline significant association between cough and risk of infection. Finally, Xin et al. (18) reported increased risks of transmission when the index case had severe disease, relative to less severe disease.
Overall, while there was some inconsistency across individual studies, certain characteristics of household interactions appeared to modestly, if not substantially, increase risk of COVID-19 infection, particularly prolonged contact in shared living/sleeping spaces, numerous infections within one household, and crowded living conditions. Unfortunately, many studies analyzed household transmission with different metrics and levels of granularity, making it challenging to compare the relative importance of specific household interactions in transmission risk. For example, some studies simply evaluated AR among “households” without assessing specific conditions of these household contact scenarios. Others that collected more detailed information did so in different ways—for example, only one study evaluated the effect of “sharing a bedroom” or estimated risks associated with time spent speaking with an index case (22). The other two studies assessing the duration of contact time (of any type) spent with index cases did so using different duration cutoffs (<3 days compared to ≥3 days, relative to 3–11 or ≥11 days compared to 1–2 days). It also is worth noting that most of the available studies evaluated transmission prior to the availability of vaccines for SARS-CoV-2 and were largely conducted in China and other Asian countries with specific infection control policies that affect transmission likelihood, thus potentially limiting generalizability to other countries.
Community and social settings
Multiple studies were identified that provided information on SARS-CoV-2 infection rates arising from community and social activities. Seven studies discussed various aspects of community-related exposure scenarios (14,20,25-29).
In a retrospective cohort study of 67 clusters and 226 confirmed cases of COVID-19 in Sichuan Province, China, as of March 17, 2020, Mao et al. (14) reported that 18% of cases were associated with public places and 12% with multiple places.
Marshall et al. (27) conducted a survey of 364 COVID-19 patients diagnosed in Colorado in March 2020; only 99 (27%) patients reported contact with a person with COVID-19. Among 265 (73%) patients without a known contact with a laboratory-confirmed COVID-19 patient, only a third (30%; 79 of 265) noted they had contact with a person with fever or respiratory symptoms. Commonly reported activities in the two weeks before being diagnosed included the following: attending gatherings larger than 10 persons (116; 44%), traveling in the US (76; 29%), working in a health care setting (75; 28%), visiting a health care setting not as a health care worker (61; 23%), and using public transportation (57; 22%).
Ravindran et al. (29) reported on a retrospective cohort of 41 wedding attendees in March 2020 in India. 56% (n=23) of the attendees developed COVID-19 in the two weeks following the wedding, resulting in a cluster of cases. Moreover, the authors reported one secondary case in a household contact of a wedding attendee.
Noh et al. (28) performed a retrospective cohort study of 76 patients with COVID-19 and 148 close contacts (n=244) and evaluated various non-household exposure scenarios. A non-household contact of having a face-to-face conversation, eating together, or using the same toilet increased the odds of infection by around seven-fold.
Fisher et al. (25) conducted a case-control study of 314 symptomatic patients who actively sought testing during July 1–29, 2020, at 11 health care facilities. The authors evaluated several community exposure scenarios 14 days prior to onset of illness, such as shopping, gatherings in the home, office setting, salon, gym, public transportation, bar/coffee shop, and church/religious gathering. The only significant increased odds were found for eating indoors or outdoors at a restaurant (OR =2.37; 95% CI: 1.49–3.78). According to the authors, wearing a mask most or all of the time significantly reduced the odds of infection in restaurant and bar settings (P=0.03 and P=0.01, respectively), but a corresponding effect estimate was not provided.
Goncalves et al. (26) performed a population-based case-control study of 271 cases and 1,396 controls from Porto Alegre, Brazil, and evaluated transmission associated with different categories of routine activities. Compared with going out every day for work or regular activity, going out every day for some activity, going out from time to time, going out just for essential tasks, and staying home all the time all reduced the odds of developing COVID-19 by ~50–70%. Social distancing moderately or more also reduced the odds of developing COVID-19 by ~70%, while mask use yielded the greatest odds reduction (by 90%).
Sami et al. (20) conducted a cross-sectional study that evaluated work- and community-related factors for 466 staff who worked in a FEMA building. None of the community-related factors resulted in a significant difference between COVID-19-positive and -negative participants. The community factors included traveling by bus, train, or subway, traveling by taxi or rideshare, attending social gatherings of more than 50 persons, and visiting a healthcare facility.
Two studies included information from a school setting. In a prospective cohort study of 181 volunteers (53 children and 118 adults) conducted by Meuris et al. (30), 45 positive cases occurred, and there was no statistically significant difference in the infection rate between adults and children. A reconstruction of the transmission of infection suggested that a majority of infection transmission events were between teachers and between children within the school. Fontanet et al. (31) reported on a retrospective cohort study of 661 participants among pupils, parents, siblings, and staff in Oise, France, between March 29 and April 4, 2020. The study evaluated the SARS-CoV-2 AR in various groups according to their status at the high school. The AR was highest in school staff (59.3%; 16 of 27 infected), teachers (43.4%; 23 of 53 infected) and students (38.3%; 92 of 240 infected), while parents (11.4%) and siblings (10.2%) had the lowest ARs.
Three additional studies were identified that evaluated infection scenarios in airline passengers. Zhang et al. (32) conducted a retrospective cohort study of 4,492 passengers and crew bound for Beijing Capital International Airport in March 2020 that were screened upon arrival for symptoms or COVID-19 infection. Approximately 130,000 total passengers arrived at the airport in the month. Of the 4,492-person screening cohort, 161 confirmed cases of COVID-19 were documented. The masked per person infection risk was reported as 0.56% (0.41–0.72%) for a 13-hour flight in economy class and 2.2% (no CI reported) for a 13-hour flight in first class. Blomquist et al. (33) conducted a retrospective cohort study of 2,313 aircraft passengers on 45 international flights from England during the first quarter of 2020. The authors evaluated sitting within two seats of an index case, household, or other travel contact with an index case. The AR for contact-traced cases within two-seats of flight-only contacts was 3.8% compared to 50% for multiple-exposure contacts sitting within two seats. Hu et al. (34) evaluated 175 COVID-19 cases among 5,797 passengers on 177 airplanes traveling from Wuhan before the lockdown on January 23, 2020. The AR ranged from 0.33% to 0.60% for traveling from Wuhan. Increasing travel time and sitting in seats adjacent to the index cases increased the AR (e.g., AR of 1.30% for adjacent seats vs. 0.04% for 3 rows away).
One additional study was identified on infection rates in cruise ships. Plucinski et al. (35) conducted a survey of 229 American passengers on the Diamond Princess cruise ship. One hundred fourteen (n=114) of the total of 437 American passengers (26%) tested positive for COVID-19. Of the 229 participating in the survey, the AR was highest among those with a symptomatic infected cabinmate (81%), 63% for those with an asymptomatic infected cabinmate, and 18% for those without an infected cabinmate.
Overall, while the number of studies was limited and the analyses used varying metrics for exposure, six of the seven studies found that community-related exposure scenarios led to higher SARS-CoV-2 infection rates than other scenarios (14,25-29). Both of the studies in school settings suggested the majority of the transmissions occurred within the school (30,31). The single study on the occurrence in a cruise ship resulted in the highest infection rate in those with a symptomatic cabinmate (35), a scenario that is similar to and consistent with a household exposure scenario. Finally, the three studies on transmission during flights indicated very low infection rates (10,32-34).
Occupational settings
The majority of studies in the literature that have evaluated work-related transmission of SARS-CoV-2 have focused on occupational exposure among healthcare workers, where contact with SARS-CoV-2 and other infectious agents can be expected due to the occupational interaction with infected individuals. However, as previously noted, healthcare settings and other occupations at the “front lines” where encounters with individuals infectious with any microbial agent (possibly unrecognized or unknown) and where protective equipment and specific precautions should be in place, were outside of the scope of this review. Of the studies reviewed, a small number directly provided information regarding the probability or risk of transmission of COVID-19 within a non-healthcare workplace, and very few attempted to quantify these risks.
During the early phases of the pandemic, certain industries experienced outbreaks more frequently than others, particularly those that either chose to remain open or were deemed essential. For example, in a study that analyzed 199 workplace outbreaks by industry sector in Ontario, Canada, from January to June 2020, it was reported that 68% of the outbreaks (and 80% of cases) occurred in just three industry sectors: (I) manufacturing, (II) agriculture, forestry, fishing, and hunting, and (III) transportation and warehousing (36). Numerous studies have also been conducted of large outbreaks that were observed in the worker populations of meat and food processing plants around the world.
Herstein et al. (37) performed a cohort study of approximately 26,000 workers at 13 meat processing plants in Nebraska between April 1 and July 31, 2020 and reported that 5,002 workers were diagnosed with COVID-19, corresponding to an AR of 19%. Despite this, the authors noted that they were unable to determine if transmission occurred in the workplace or elsewhere in the community. Mallet et al. (38) performed a retrospective study of a COVID-19 outbreak among 1,347 workers in a French pork processing plant, in which 140 occupational cases were identified in the deboning and cutting department, corresponding to an AR of 16.6% and a statistically significantly elevated risk of 3.68 (95% CI: 2.41–5.64; P<0.001) compared to workers in other departments. However, it should be noted that the authors of the study reported that over half of the cases (n=62) reported carpooling or sharing their accommodation with one or more other workers, without specifying whether they were family members, potentially implicating household or community transmission. Furthermore, Mora et al. (21) conducted a cross-sectional study of risk factors for 1,107 farmworkers in Monterey County, California, in which a 4-fold increase in positive COVID-19 tests among farmworkers in community clinics between June and November 2020 than in the county population at large (22% vs. 6%). The authors reported that the incidence of COVID-19 among workers was elevated among those who had a documented exposure to a known or suspected case of COVID-19 in the workplace (RR =1.59; 95% CI: 1.18–2.14); however, it is unclear if this scenario is representative of the overall risk of the workforce evaluated. Notably, it was also reported that incidence was elevated based on several non-work-related factors, such as education, ethnicity, exposure to COVID-19 in the household, and other health conditions. Indeed, it has been theorized that several non-work-related socioeconomic factors may have influenced these outbreaks, including the presence of a relatively young workforce who are more likely to be asymptomatic, the workforce’s reliance on crowded housing and transportation, and job insecurity that discourages workers from reporting symptoms (39).
In addition, the likelihood of transmission appears to be elevated in occupations requiring interaction with the general public, such as taxi drivers, grocery store workers, and school staff, for example, compared to settings that do not require these interactions. Lan et al. (40) performed an observational study of 103 work-related cases from six Asian countries or regions, including Hong Kong, Japan, Singapore, Taiwan, Thailand, and Vietnam, to identify the occupations at higher risk of COVID-19 transmission. Based on their analysis, they identified high-risk occupations as car, taxi and van drivers, shop salespersons, domestic housekeepers, religious professionals, construction laborers, and tour guides. Notably, the authors reported that, in the early transmission period, defined as the first 10 days from when the first locally transmitted case was reported, 48% of cases were due to work-related exposure, while in the late transmission period, defined as the 11th to 40th day of the study period, only 11% were work-related, a statistically significant decrease (P<0.0001). In addition, Lan et al. (41) performed a cross-sectional study that investigated infection and exposure risks among 104 grocery retail workers who tested positive for COVID-19 in May of 2020 in Massachusetts. The authors reported that employees with direct customer exposure (such as cashiers, front end associates, sales associates, fresh food associates, cart attendants, janitorial crew, supervisors, and managers) had a statistically significantly increased odds (OR =5.1; 95% CI: 1.1–24.8) of testing positive compared to workers with no direct customer exposure, such as stockers, backroom workers, receiving workers, and maintenance workers.
Ng et al. (22) performed a retrospective cohort study of 7,770 close contacts of COVID-19 cases in Singapore between January 23 and April 3, 2020, including 2,231 work contacts. Work contacts were defined as individuals who came into close contact (within 2 meters for at least 30 minutes) with the index case at work from 2 days before the onset of symptoms to isolation of the case. Among work contacts, the SAR was 1.3% (95% CI: 0.9–1.9%), which was lower than the SARs among household contacts (5.5%), indicating a higher risk of transmission in the household compared to the workplace. Based on an evaluation of 466 FEMA office workers between March and October of 2020, Sami et al. (20) reported that 15 (3.2%) workers tested positive for COVID-19 antibodies. The study participants reportedly spent an average of 20.5 days in the office buildings. While the authors found no significant difference in use of mitigation activities, such as the use of masks and/or hand sanitizer and social distancing, they observed that a significantly increased percentage of negative cases lived with someone who had a positive test for SARS-CoV-2. These results indicate that these workers were more likely to contract COVID-19 in their own household versus at work.
Fontanet et al. (31) performed a retrospective cohort study of 661 individuals in a French high school setting from March 30 to April 4, 2020. Among school staff, 16 of 27 tested positive for COVID-19, corresponding to an infection AR of 59.3%. Similarly, among teachers, 23 of 53 tested positive, corresponding to an infection AR of 43.4%. Both of these ARs were higher than the ARs among pupils (38.3%), parents (11.4%), and siblings (10.2%). In addition, Meuris et al. conducted a prospective cohort study of 181 volunteers (53 children and 118 adults), in which 45 cases of COVID-19 were identified. The authors reported that, based on a reconstruction of the transmission of infection, a majority of infection transmission events were between teachers and between children within the school.
Chaw et al. (8) conducted a cluster investigation of 848 workplace/school close contacts of confirmed COVID-19 cases in Brunei. The authors reported that, among 848 close contacts in the workplace or school, only 6 individuals tested positive for COVID-19, corresponding to an AR of 0.7% (95% CI: 0.3–1.6%) and a non-significant risk ratio of 0.79 (95% CI: 0.23–3.10) relative to close contacts encountered in a social setting. Zhang et al. [2020] (42) analyzed contact-tracing data collected in Guangzhou, China, between January 28 and March 15, 2020 for presymptomatic COVID-19 cases. The authors reported a SAR of 0.0 for workplace transmission based on zero cases identified out of 119 close contacts. The authors theorized that the low transmission level they reported could be the result of active surveillance, centralized quarantine, and forceful social-distancing strategies in Guangzhou, China, during the time period of the study.
Park et al. (15) evaluated an outbreak in which 97 COVID-19 cases were documented in March of 2020 out of 1,143 employees of a call center in South Korea. Of the 97 cases, 94 worked on the same floor of the building out of a total of 216 employees, corresponding to an AR of 43.5% (95% CI: 36.9–50.4%). It should be noted that this outbreak occurred early in the pandemic, and the workers on the 11th floor were in close quarters, which facilitated a high degree of transmission. The authors reported that contact tracing, testing, and quarantine limited further transmission.
Finally, in the study of European hospital workers conducted by Kahlert et al. (12), it was reported that weekly (OR =2.98; 95% CI: 1.47–6.68; P=0.001) and daily (OR =2.54; 95% CI: 1.29–5.57; P=0.004) frequency of meals in a staff lunchroom increased the odds of infection compared to never frequency, while occasional frequency did not increase the risk of infection compared to never frequency, which potentially implicates the behavior of employees during breaks or time off of work as an influential factor affecting SARS-CoV-2 transmission.
In summary, early in the pandemic (i.e., January through March of 2020), when little information regarding SARS-CoV-2 was available, transmission rates were elevated in industries that remained open with minimal exposure controls present or in settings involving employees who worked in close quarters, such as meat-processing facilities or call centers. However, information on community reproduction rates were not reported preventing the ability to draw firm conclusions. In other workplace settings where exposure controls may or may not have been implemented, SARS-CoV-2 ARs were no different from other settings with similar degrees of interpersonal contact.
Discussion
The nature of the COVID-19 pandemic has necessitated the rapid conduct and dissemination of studies informing potential transmission and control of this highly infectious virus (43). One consequence of “real-time” reporting of data is that many available studies are subject to numerous weaknesses, including variable study designs, reliance on surveys that are prone to information biases (e.g., recall bias, reporting bias), selection biases (e.g., nonresponse, awareness bias), minimal follow-up time, and the inability to identify and address potential confounding factors. These limitations extend to this review and introduce uncertainties in drawing conclusions regarding the most critical settings and characteristics associated with COVID-19 transmission.
Furthermore, many factors influence the likelihood and duration of exposure to SARS-CoV-2, including the mode of transmission, which further complicates the ability to draw conclusions regarding the origin of infection. For example, while direct droplet transmission is largely dependent on short range proximity (<6 feet) to an infectious individual, airborne transmission can occur over longer distances, depending on the size of the respiratory droplet, the number and proximity of infectious individuals, and environmental factors, such as room size, air changes per hour, air distribution patterns, room pressurization, temperature, humidity, percent outdoor air, and air filtration/treatment (e.g., ultraviolet germicidal irradiation) (44). Fomite transmission, which occurs when individuals are exposed to the virus from touching surfaces contaminated by settled respiratory secretions or droplets from infected individuals, followed by touching the mouth, nose, or eyes, is governed by human behaviors (e.g. hand hygiene and face touching) and the likelihood for contact with contaminated surfaces (a function of the frequency with which surfaces are touched, cleaning and disinfection efficacy, residence time of SARS-CoV-2 on surfaces, and glove-wearing practices). As such, numerous combinations of exposure factors can occur within each setting, and the infection risk posed to an individual in any given exposure scenario will depend on the number of risk factors present. Therefore, estimating the relative magnitude of effect of any one of these factors for an individual is challenging.
Despite these challenges, the available studies provide valuable information on settings and specific factors within these settings that are most likely to increase individuals’ risk of contracting SARS-CoV-2. We were able to identify some fairly consistent patterns across the studies reviewed, which are strengthened by both existing and evolving understanding of environmental, structural (i.e., building characteristics), and virological factors that affect the survival and transmissibility of SARS-CoV-2. Specifically, this body of literature suggests that the household setting is associated with the highest secondary transmission ARs. Several studies (7,9,22,25) compare the relative AR among household, workplace, and “non-household” settings (gatherings, grocery stores, gyms, salons, transportation, etc.) (7,9,22,25). Given the sustained and frequent contact with those within the household, the possibility for low air exchange rates, and considering that most families do not wear masks around one another, this result is not surprising. The body of literature on social/community settings was more limited (particularly for schools), but most studies reported that attending large gatherings, traveling, visiting a health care setting, and using public transportation led to higher transmission risk, relative to avoidance of such activities. While each setting led to some level of secondary transmission, household transmission typically predominated and almost always predominated when accompanied by high community transmission.
Our conclusions are similar to those reported in Thompson et al. (16), a systematic review and meta-analysis of setting-specific transmission rates. The authors employed several sophisticated models to account for both within-study correlations from local homogeneity in interventions and home, travel, and work practices, as well as between-study heterogeneity stemming from differences across studies and populations. Thompson et al. (16) reported pooled SARs of 21.1% for households (95% CI: 17.4–24.8%), 1.9% for workplaces (95% CI: 0.0–3.9%), 3.6% (95% CI: 1.0–6.9%) for healthcare facilities, 1.2% (95% CI: 0.3–2.1%) for social settings with casual contacts/strangers, and 5.9% (95% CI: 3.8–8.1%) for social settings with family and friends. While the highest pooled SARs occurred in household settings, the highest R0 was for healthcare settings, likely due to high number of contacts per individual. Further, Thompson et al. (16) conducted sensitivity analyses, indicating that isolation of index cases within 5 days of symptom onset reduced both SAR and R0.
Teasing out the independent effect of workplace transmission risk within a complex blend of environmental and human factors is challenging. Evidence has demonstrated that those who work within sectors that have remained active during the pandemic have increased risk of infection, hospitalization, and/or premature death from COVID-19. This risk may be exacerbated in occupations that require individuals to commute to a workplace compared with occupations that support remote work options. Based on the evidence reviewed in this paper, there is a clear interaction between household, community, and occupational risk of transmission of SARS-CoV-2, which in turn may encompass and/or correlate with a multitude of other factors, including personal behaviors (hand hygiene, use of masks) and environmental/socioeconomic factors, such as crowded housing, job insecurity, and poverty (45). When evaluating safety protocols or the cause(s) of previous clusters of cases of COVID-19 in the workplace, it is challenging to determine the relative contribution of transmission in the workplace relative to community (and transportation) or household exposures.
Our research supports the approach taken by Occupational Safety and Health Administration (OSHA), which classifies risk of worker exposure based principally on the likelihood of close contact with others within or outside of the workplace who may be infected with SARS-CoV-2 into the following stratified exposure groups (45):
- Lower exposure risk: jobs that do not require close contact with others and who have minimal occupational contact with the community members, such as remote workers and office workers who do not frequently work with others.
- Medium exposure risk: jobs that require frequent and/or sustained close contact with others outdoors or well-ventilated areas, such as those working closely with coworkers, and those who have frequent contact with the community, such as retail workers, transit workers, law enforcement and emergency response workers, and restaurant/bar workers.
- High exposure risk: jobs with a high exposure potential to known or suspected sources of COVID-19, such as healthcare staff who enter patient rooms, medical transport works, mortuary workers, those who have frequent and/or sustained close contact with others indoors or in poorly-ventilated areas, such as those working closely with coworkers, and those who have frequent contact with the community, such as retail workers, transit workers, law enforcement and emergency response workers, and restaurant/bar workers.
- Very high exposure risk: jobs with very high exposure potential to known or suspected cases of COVID-19 during medical, postmortem, or laboratory procedures, such as healthcare workers, laboratory personnel, or morgue workers.
Furthermore, while behaviors in the household and community are largely unregulated, the workplace is regulated under the Occupational Safety and Health Administration (OSHA), which mandates that “employers are responsible for providing a safe and healthy workplace free from recognized hazards likely to cause death or serious physical harm” (46,47). Within the field of industrial hygiene, a hierarchy of controls is used to determine how to minimize or eliminate employee exposures to hazards in the workplace. In order of decreasing effectiveness, hazard control strategies include elimination (i.e., physically removing the hazard), substitution (i.e., replacing the hazard), engineering controls (i.e., isolating people from the hazard), administrative controls (i.e., changing the way people work), and PPE (i.e., protecting the worker with PPE) (48). While elimination of a hazard is the most desirable method for controlling exposures, it is difficult to achieve for a viral hazard, which requires an employer to implement a combination of the lower-tier control strategies to limit exposure. While this framework was developed to mitigate occupational hazards and risk, it can also be used in the workplace to mitigate exposure to community-based infectious hazards, such as infectious agents.
Indeed, OSHA and others have provided control recommendations that employers should consider to mitigate the spread of SARS-CoV-2 in the workplace (46,49). These include, but are not limited to, keeping susceptible or symptomatic individuals at home (elimination; administrative control), maintaining ventilation systems (engineering control), physical distancing (administrative control), making face-coverings or respirators (if applicable) available (administrative control and PPE), education and training regarding COVID-19 policies and procedures (administrative control), and facilitating vaccination (administrative control). The effects of implementation of one or multiple of these controls were shown to reduce transmission in a workplace. For example, Herstein et al. (37) evaluated the effectiveness of different controls for reducing incidence of COVID-19 in a Nebraska meat-processing facility and reported that there was a significantly reduced incidence of COVID-19 cases in 62% of studied facilities following adoption of universal masking and physical barrier interventions.
Recently, Baptista et al. (50) developed a semi-quantitative framework for assessing risk of COVID-19 morbidity and mortality and recommended mitigation strategies. Baptista et al. (50) developed three matrices of recommended control strategies, which are derived by first considering community transmission using the WHO metrics (magnitude of new cases, percentage of positive tests, and daily new cases per 100,000 people), the strata of OSHA occupational risk summarized above, and presence of individual risk factors for severe disease (numerous pre-existing conditions). Recommended workplace practices are based on the estimated risk of transmission. These strategies range from “standard” (hand hygiene, respiratory protection, 6 feet or more of distance, including in cafeterias) to full exclusion (work from home, sick leave/other leave).
Ultimately, it is difficult to determine the exact level of transmission for each workplace and how to parse out the influence of social/community and household exposures on spread within the workplace. However, the information presented in this paper, coupled with frameworks provided by other agencies and authors, should allow employers to evaluate, at a high-level, the overall likelihood of transmission of SARS-CoV-2 (or other similarly highly infectious viruses) within their workplace, and evaluate and implement risk management strategies to control infections, to the greatest extent possible.
Acknowledgments
The authors would like to thank Dr. Natalie Egnot for her review of this manuscript and Carrie Kahn for her review and assistance in preparing this manuscript.
Funding: None.
Footnote
Provenance and Peer Review: This article was commissioned by the editorial office, Journal of Public Health and Emergency for the series “Managing COVID-19 Risks in the Workplace”. The article has undergone external peer review.
Reporting Checklist: The authors have completed the Narrative Review reporting checklist. Available at https://jphe.amegroups.com/article/view/10.21037/jphe-22-12/rc
Conflicts of Interest: All authors have completed the ICMJE uniform disclosure form (available at https://jphe.amegroups.com/article/view/10.21037/jphe-22-12/coif). The series “Managing COVID-19 Risks in the Workplace” was commissioned by the editorial office without any funding or sponsorship. All of the authors are or were employed by Cardno ChemRisk, a consulting firm that provides scientific advice to the government, corporations, law firms, and various scientific/professional organizations. Cardno ChemRisk scientists have been engaged by numerous organizations to provide COVID-related toxicology, epidemiology, and industrial hygiene health and safety advice. EB is now employed by Benchmark Risk Group, but all work performed on this manuscript occurred during his employment at ChemRisk (Stantec). HNL and SHG have served as contributors to the AIHA Back to Work Safely Task Force by drafting industry-specific guidance for businesses and consumers to safely reopen after emerging from COVID-19 shelter-in-place/quarantine. HNL and SHG, among others at ChemRisk, received the American Industrial Health Association’s Social Responsibility and President’s Awards for our work on COVID-19 with the Task Force. The time invested by these authors to write this article was provided by their employer. KAM serves as an unpaid Guest Editor of the series and serves as an unpaid editorial board member of Journal of Public Health and Emergency from October 2021 to September 2023. KAM also provides COVID-19 preparation planning and litigation support. The authors have no other conflicts of interest to declare.
Ethical Statement: The authors are accountable for all aspects of the work in ensuring that questions related to the accuracy or integrity of any part of the work are appropriately investigated and resolved.
Open Access Statement: This is an Open Access article distributed in accordance with the Creative Commons Attribution-NonCommercial-NoDerivs 4.0 International License (CC BY-NC-ND 4.0), which permits the non-commercial replication and distribution of the article with the strict proviso that no changes or edits are made and the original work is properly cited (including links to both the formal publication through the relevant DOI and the license). See: https://creativecommons.org/licenses/by-nc-nd/4.0/.
References
- WHO. Technical Guidance: Naming the Coronavirus Disease (COVID-19) and the Virus that Caused It. Retrieved Jan 27, 2022. Available online: https://www.who.int/emergencies/diseases/novel-coronavirus-2019/technical-guidance/naming-the-coronavirus-disease-(covid-2019)-and-the-virus-that-causes-it (Geneva: World Health Organization (WHO); 2022).
- CDC. COVID-19: Scientific Brief-SARS-COV-2 Transmission: Summary of Recent Changes. Updated May 7, 2021. Retrieved Jan 27, 2022. Available online: https://www.cdc.gov/coronavirus/2019-ncov/science/science-briefs/sars-cov-2-transmission.html (Atlanta, GA: Centers for Disease Control and Prevention (CDC); 2021).
- Singanayagam A, Hakki S, Dunning J, et al. Community transmission and viral load kinetics of the SARS-CoV-2 delta (B.1.617.2) variant in vaccinated and unvaccinated individuals in the UK: a prospective, longitudinal, cohort study. Lancet Infect Dis 2022;22:183-95. [Crossref] [PubMed]
- CDC. Omicron Variant: What You Need to Know. Centers for Disease Control and Prevention. U.S. Department of Health & Human Services. 2021. Available online: https://www.cdc.gov/coronavirus/2019-ncov/variants/omicron-variant.html (Accessed February 2, 2022).
- Sanche S, Lin YT, Xu C, et al. High Contagiousness and Rapid Spread of Severe Acute Respiratory Syndrome Coronavirus 2. Emerg Infect Dis 2020;26:1470-7. [Crossref] [PubMed]
- TIME. A COVID-Free Pacific Nation Opened Its Border a Crack. The Virus Came Rushing In. 2022. Available online: https://time.com/6143260/covid-19-pacific-islands-kiribati/ (Accessed February 2, 2022).
- Burke RM, Calderwood L, Killerby ME, et al. Patterns of Virus Exposure and Presumed Household Transmission among Persons with Coronavirus Disease, United States, January-April 2020. Emerg Infect Dis 2021;27:2323-32. [Crossref] [PubMed]
- Chaw L, Koh WC, Jamaludin SA, et al. Analysis of SARS-CoV-2 Transmission in Different Settings, Brunei. Emerg Infect Dis 2020;26:2598-606. [Crossref] [PubMed]
- Ehrhardt J, Ekinci A, Krehl H, et al. Transmission of SARS-CoV-2 in children aged 0 to 19 years in childcare facilities and schools after their reopening in May 2020, Baden-Wurttemberg, Germany. Euro Surveill 2020;25:2001587. [Crossref] [PubMed]
- Ge Y, Martinez L, Sun S, et al. COVID-19 Transmission Dynamics Among Close Contacts of Index Patients With COVID-19: A Population-Based Cohort Study in Zhejiang Province, China. JAMA Intern Med 2021;181:1343-50. [Crossref] [PubMed]
- Han T. Outbreak investigation: transmission of COVID-19 started from a spa facility in a local community in Korea. Epidemiol Health 2020;42:e2020056. [Crossref] [PubMed]
- Kahlert CR, Persi R, Güsewell S, et al. Non-occupational and occupational factors associated with specific SARS-CoV-2 antibodies among hospital workers - A multicentre cross-sectional study. Clin Microbiol Infect 2021;27:1336-44. [Crossref] [PubMed]
- Li Y, Liu J, Yang Z, et al. Transmission of Severe Acute Respiratory Syndrome Coronavirus 2 to Close Contacts, China, January-February 2020. Emerg Infect Dis 2021;27:2288-93. [Crossref] [PubMed]
- Mao S, Huang T, Yuan H, et al. Epidemiological analysis of 67 local COVID-19 clusters in Sichuan Province, China. BMC Public Health 2020;20:1525. [Crossref] [PubMed]
- Park SY, Kim YM, Yi S, et al. Coronavirus Disease Outbreak in Call Center, South Korea. Emerg Infect Dis 2020;26:1666-70. [Crossref] [PubMed]
- Thompson HA, Mousa A, Dighe A, et al. Severe Acute Respiratory Syndrome Coronavirus 2 (SARS-CoV-2) Setting-specific Transmission Rates: A Systematic Review and Meta-analysis. Clin Infect Dis 2021;73:e754-64. [Crossref] [PubMed]
- Wu J, Huang Y, Tu C, et al. Household Transmission of SARS-CoV-2, Zhuhai, China, 2020. Clin Infect Dis 2020;71:2099-108. [Crossref] [PubMed]
- Xin H, Jiang F, Xue A, et al. Risk factors associated with occurrence of COVID-19 among household persons exposed to patients with confirmed COVID-19 in Qingdao Municipal, China. Transbound Emerg Dis 2021;68:782-8. [Crossref] [PubMed]
- Hu S, Wang W, Wang Y, et al. Infectivity, susceptibility, and risk factors associated with SARS-CoV-2 transmission under intensive contact tracing in Hunan, China. Nat Commun 2021;12:1533. [Crossref] [PubMed]
- Sami S, Vuong N, Miller H, et al. SARS-CoV-2 Infection and Mitigation Efforts among Office Workers, Washington, DC, USA. Emerg Infect Dis 2021;27:669-72. [Crossref] [PubMed]
- Mora AM, Lewnard JA, Kogut K, et al. Risk Factors Associated With SARS-CoV-2 Infection Among Farmworkers in Monterey County, California. JAMA Netw Open 2021;4:e2124116. [Crossref] [PubMed]
- Ng OT, Marimuthu K, Koh V, et al. SARS-CoV-2 seroprevalence and transmission risk factors among high-risk close contacts: a retrospective cohort study. Lancet Infect Dis 2021;21:333-43. [Crossref] [PubMed]
- Ghosh AK, Venkatraman S, Soroka O, et al. Association between overcrowded households, multigenerational households, and COVID-19: a cohort study. Public Health 2021;198:273-9. [Crossref] [PubMed]
- Jashaninejad R, Doosti-Irani A, Karami M, et al. Transmission of COVID-19 and its Determinants among Close Contacts of COVID-19 Patients Running title. J Res Health Sci 2021;21:e00514. [Crossref] [PubMed]
- Fisher KA, Tenforde MW, Feldstein LR, et al. Community and Close Contact Exposures Associated with COVID-19 Among Symptomatic Adults ≥18 Years in 11 Outpatient Health Care Facilities - United States, July 2020. MMWR Morb Mortal Wkly Rep 2020;69:1258-64. [Crossref] [PubMed]
- Gonçalves MR, Dos Reis RCP, Tólio RP, et al. Social Distancing, Mask Use, and Transmission of Severe Acute Respiratory Syndrome Coronavirus 2, Brazil, April-June 2020. Emerg Infect Dis 2021;27:2135-43. [Crossref] [PubMed]
- Marshall K, Vahey GM, McDonald E, et al. Exposures Before Issuance of Stay-at-Home Orders Among Persons with Laboratory-Confirmed COVID-19 - Colorado, March 2020. MMWR Morb Mortal Wkly Rep 2020;69:847-9. [Crossref] [PubMed]
- Noh JY, Song JY, Hyun HJ, et al. Risk factors for SARS-CoV-2 transmission in non-household clusters. J Infect 2021;83:e22-4. [Crossref] [PubMed]
- Ravindran B, Hogarth F, Williamson K, et al. High COVID-19 attack rate among attendees of wedding events in Bali, Indonesia, March 2020. Commun Dis Intell 2018;2020.
- Meuris C, Kremer C, Geerinck A, et al. Transmission of SARS-CoV-2 After COVID-19 Screening and Mitigation Measures for Primary School Children Attending School in Liège, Belgium. JAMA Netw Open 2021;4:e2128757. [Crossref] [PubMed]
- Fontanet A, Tondeur L, Madec Y, et al. Cluster of COVID-19 in northern France: A retrospective closed cohort study. Lancet 2020; [Crossref]
- Zhang J, Qin F, Qin X, et al. Transmission of SARS-CoV-2 during air travel: a descriptive and modelling study. Ann Med 2021;53:1569-75. [Crossref] [PubMed]
- Blomquist PB, Bolt H, Packer S, et al. Risk of symptomatic COVID-19 due to aircraft transmission: a retrospective cohort study of contact-traced flights during England's containment phase. Influenza Other Respir Viruses 2021;15:336-44. [Crossref] [PubMed]
- Hu M, Wang J, Lin H, et al. Risk of Severe Acute Respiratory Syndrome Coronavirus 2 (SARS-CoV-2) Transmission Among Air Passengers in China. Clin Infect Dis 2022;75:e234-40. [Crossref] [PubMed]
- Plucinski MM, Wallace M, Uehara A, et al. Coronavirus Disease 2019 (COVID-19) in Americans Aboard the Diamond Princess Cruise Ship. Clin Infect Dis 2021;72:e448-57. [Crossref] [PubMed]
- Murti M, Achonu C, Smith BT, et al. COVID-19 Workplace Outbreaks by Industry Sector and Their Associated Household Transmission, Ontario, Canada, January to June, 2020. J Occup Environ Med 2021;63:574-80. [Crossref] [PubMed]
- Herstein JJ, Degarege A, Stover D, et al. Characteristics of SARS-CoV-2 Transmission among Meat Processing Workers in Nebraska, USA, and Effectiveness of Risk Mitigation Measures. Emerg Infect Dis 2021;27:1032-8. [Crossref] [PubMed]
- Mallet Y, Pivette M, Revest M, et al. Identification of Workers at Increased Risk of Infection During a COVID-19 Outbreak in a Meat Processing Plant, France, May 2020. Food Environ Virol 2021;13:535-43. [Crossref] [PubMed]
- Middleton J, Reintjes R, Lopes H. Meat plants-a new front line in the covid-19 pandemic. BMJ 2020;370:m2716. [Crossref] [PubMed]
- Lan FY, Wei CF, Hsu YT, et al. Work-related COVID-19 transmission in six Asian countries/areas: A follow-up study. PLoS One 2020;15:e0233588. [Crossref] [PubMed]
- Lan FY, Suharlim C, Kales SN, et al. Association between SARS-CoV-2 infection, exposure risk and mental health among a cohort of essential retail workers in the USA. Occup Environ Med 2021;78:237-43. [Crossref] [PubMed]
- Zhang W, Cheng W, Luo L, et al. Secondary transmission of Coronavirus Disease from presymptomatic Persons, China. Emerg Infect Dis 2020;26:1924-6. [Crossref] [PubMed]
- Hollins D, Kiorpes AL. Evaluating the industrial hygiene, toxicology, and public health aspects of COVID-19. Toxicol Ind Health 2020;36:605-6. [Crossref] [PubMed]
- Zisook RE, Monnot A, Parker J, et al. Assessing and managing the risks of COVID-19 in the workplace: Applying industrial hygiene (IH)/occupational and environmental health and safety (OEHS) frameworks. Toxicol Ind Health 2020;36:607-18. [Crossref] [PubMed]
- OSHA. COVID-19. Hazard Recognition. Retrieved Jan 27, 2022. Available online: https://www.osha.gov/coronavirus/hazards (Washington, D.C.: Occupational Safety and Health Administration (OSHA); 2022).
- OSHA. COVID-19. Protecting Workers: Guidance on Mitigating and Preventing the Spread of COVID-19 in the Workplace. Updated June 10, 2021. Retrieved Jan 27, 2022. Available online: https://www.osha.gov/coronavirus/safework (Washington, D.C.: Occupational Safety and Health Administration (OSHA); 2021).
- U.S. Congress. Occupational Safety and Health Act of 1970. Washington, D.C.: Government Printing Office. Public Law 91-5961970.
- NIOSH. Workplace Safety & Health Topics: Hierarchy of Controls. Page Last Reviewed Jan. 13, 2015. Retrieved Jan. 27, 2022. Available online: https://www.cdc.gov/niosh/topics/hierarchy/default.html (Cincinnati, OH: Centers for Disease Control and Prevention (CDC)-National Institute for Occupational Safety and Health (NIOSH); 2015).
- Sehgal NJ, Milton DK. Applying the Hierarchy of Controls: What Occupational Safety Can Teach us About Safely Navigating the Next Phase of the Global COVID-19 Pandemic. Front Public Health 2021;9:747894. [Crossref] [PubMed]
- Baptista MC, Burton WN, Pawlecki B, et al. A Physician's Guide for Workers' Return to Work During COVID-19 Pandemic. J Occup Environ Med 2021;63:199-220. [Crossref] [PubMed]
Cite this article as: Lynch HN, Beckett EM, Dobyns LL, Thompson WJ, Divis HR, Encina E, Gaffney SH, Mundt KA. Literature review of the relative importance of household, community and social, and workplace settings on the probability of COVID-19 infection. J Public Health Emerg 2022;6:35.