Filtration efficiency of makeshift material masks vs. surgical/procedure masks and/or filtering facepiece respirators: a narrative review
Introduction
More than two years since the World Health Organization (WHO)’s declaration of the COVID-19 outbreak as a global pandemic, face masks remain an important tool in reducing the spread of the SARS-CoV-2 virus (1,2). A face mask is a product that covers the wearer’s nose and mouth, although there are significant differences between different mask types (3). Barrier face coverings, such as cloth masks, have been recommended by the U.S. Centers for Disease Control and Prevention (CDC) for the general public during everyday use (4). Cloth face masks may help block large particle droplets exhaled by the wearer from reaching others while speaking, coughing, or sneezing (4). At the beginning of the COVID-19 pandemic, the public was encouraged to create their own face masks at home, reserving surgical masks and respirators for health care workers (5). However, homemade masks (e.g., loosely woven cloth products), which provide the least protection, are no longer recommended now that higher-level protection masks and respirators [e.g., well-fitting disposable surgical masks, well-fitting National Institute for Occupational Safety & Health (NIOSH)-approved respirators such as N95s] are more readily available to the general population (6). Cloth masks primarily provide source control (i.e., prevent people who have COVID-19 from spreading the virus to others) and a degree of particulate filtration to reduce the amount of inhaled particulate material, but they do not provide respiratory protection to the wearer to the same extent as with filtering facepiece respirators (FFRs), such as N95s (3,7). Notably, cloth masks have not been required to meet federal performance standards. However, in February of 2021, ASTM International (formerly known as American Society for Testing and Materials) and the NIOSH jointly developed a new Barrier Face Covering Standard (ASTM F3502-21) to establish uniform testing methods and performance criteria to allow comparison of product claims regarding filtration efficiency (FE), breathability, reuse potential, and leakage (8,9). Unlike federal regulations, the new ASTM standard serves as a recommendation and is not enforceable (9).
FE
Masks that have high FE, or the ability to filter small particles such as aerosols, may reduce the transmission of airborne viruses, such as SARS-CoV-2 (10). The FE of a material is a common metric for assessing the particle capture efficiency of a material. It is a function of (I) the ability of a single fiber to retain a particle, which is governed by diffusion, interception, impaction, and electrostatic deposition efficiencies, and (II) filter material characteristics, including porosity, fiber/yarn diameter, and thickness of the filter material (11,12). FE can be quantified by measuring average particle number concentrations (PNC) (e.g., particles per cubic centimeter (p/cc) upstream (CU) and downstream (CD) of the test material (11-13). This metric is typically reported as a percentage of particles that are captured by the filter (Eq. [1]).
The TSI 8130/8130A is mandated for measuring filter efficiency for the certification of N95 respirators by NIOSH Procedure TEB-APR-STP-0059 (14). NIOSH is the only organization that can certify N95 respirators, with strict quality assurance and performance requirements to produce conservative FE (15).
Measures of FE include particle FE (PFE), for which test procedures measure the quality of masks for filtering particles of different sizes, and bacterial FE (BFE) and viral FE (VFE), for which test procedures measure how well a material filters biological aerosols (13).
To measure PFE, the FDA recommends that manufacturers use the ASTM F2299 protocol using 0.1 µm monodisperse polystyrene latex (PSL) spheres at a face velocity between 0.5 to 25 cm/s; for BFE, the FDA recommends the ASTM F2101 protocol using 3 µm aqueous Staphylococcus aureus aerosol droplets at an air flow rate of 28 L/min (face velocity not defined), and maintained at 1,700–3,000 viable colony-forming units (CFU) per test (13,16-18). Face velocity (v) permits comparison of filter measurements that use different areas, expressed as a mask-averaged speed in which the flow (Q) is distributed over the active filter material area (A) (Eq. [2]) (19).
Although there is no recognized standard VFE method, a VFE method has been created by Nelson Laboratories utilizing a modified ASTM F2101 protocol; VFE testing follows the same procedure as that for BFE, with the use of a 27 nm bacteriophage phiX174 challenge organism maintained at 1,100–3,300 plaque-forming units (PFU) per test (13,16,20). Further, the NIOSH sodium chloride (NaCl) aerosol challenge is a widely accepted method for evaluating FE of respirators that must be prequalified before submitting to NIOSH for certification; this method uses charge-neutralized NaCl particles with a count median diameter of 0.075±0.020 µm, flow rate of 85 L/min, and face velocity of 9.3 cm/s (13,14,21,22). Additionally, under the U.S. Government’s Approval of Respiratory Devices (42 CFR Part 84), NIOSH considers protection against oils when approving non-powered air-purifying respirators, which fall into three categories: N-series (Not resistant to oil), R-series (somewhat Resistant to oil), and P-series (strongly resistant, or oil Proof), at 95%, 99%, and 99.97% FE, respectively (21,23).
Penetration efficiency
Penetration efficiency (PE) is a measure of the quantity of particles that are able to pass through the filter, and can be represented by Eq. [3] (11,12,21).
Leakage
Notably, in studies assessing the performance of filtering materials, FE and PE are often quantified under idealized laboratory conditions, in which leaks are absent. However, leakage can substantially decrease the performance of any material intended to be used as a barrier or for respiratory protection (24). Leakage is the fraction of particles that pass through gaps in the interface between the mask and the face (25). As such, the total PNC fraction outside the mask is a function of PE and leakage (25).
Usage, performance, and availability of different mask types
Surgical masks (widely used in healthcare settings, and may also be referred to as isolation, dental, or medical procedure masks), regulated by the Food and Drug Administration (FDA) under 21 CFR 878.4040, are disposable devices meant to help block wearer emissions (e.g., large-particle droplets, splashes, sprays, or splatters that may contain viruses and bacteria) and also primarily provide source control. Surgical masks vary in shape and thickness, are loose-fitting, and do not filter very small particles; therefore, they are not expected to provide complete protection from airborne or aerosolized particles (7,26,27). Surgical masks filter approximately 95% to 98% of particles depending on their ASTM categorization, with Levels 1, 2, and 3 ranking from least to most effective (13).
In contrast, FFRs such as N95 respirators provide close facial fit and efficient filtration of airborne particles. N95 respirators are regulated by NIOSH and are required to filter at least 95% of non-oily airborne particles. It should be noted that the NIOSH certification testing for N95 respirators is considered the more stringent method compared to the ASTM method for surgical masks, and the ASTM 95% efficiency is not the same as the NIOSH 95% efficiency. Due to their protective capabilities, N95s have played a crucial role in protecting healthcare workers, first responders, and various essential workers on the front lines of the COVID-19 pandemic (4,7,27). However, in response to shortages of surgical masks and respirators early on in the pandemic, prioritization of these devices for essential workers, and mask requirements for the general public, cloth masks have become a research focus area (28).
While cloth masks are economical and accessible, they are not as effective at filtering particles as surgical masks and FFRs (29,30). Furthermore, various textile materials have been promoted for use as cloth masks with limited evidence and lack of standard specifications for optimal performance (28). Prior to the establishment of ASTM F3502-21 for barrier face coverings, there were no specific standards for testing non-medical face masks used by the comparatively lower-risk general public. As such, previous research on the FE of non-medical face masks comprised of a broad range of materials have been conducted using a wide variety of non-standardized testing methods, which results in challenges for quantitively comparing FE of makeshift material masks across different studies.
Study objective
To this day, face masks remain important and relevant; states, cities, and counties continue to modify face mask requirements depending on COVID-19 statistics, such as case counts. In light of highly-transmissible variants of the SARS-CoV-2 virus (e.g., Omicron), medical experts have recommended that the general public upgrade to an N95 or similar high-filtration respirator in public indoor spaces (31). As of January of 2022, the CDC clarified that cloth masks frequently worn by the general public do not provide as much protection as surgical masks or respirators (6). However, due to their reusability, ease of procurement, visual appeal, and change in cultural perception, cloth masks are likely to continue to be popular to use for years to come. Therefore, this narrative review will (I) provide a summary of the existing literature on FE of masks fabricated using readily available materials within a common household (e.g., cloth, rags, t-shirts, bedsheets, towels, bandanas, etc.), as well as combinations of different mask types worn together (e.g., surgical/procedure mask worn with a makeshift material mask), compared to surgical/procedure mask (e.g., surgical mask, procedure mask, pediatric face mask) and/or FFR controls (e.g., N95; KN95; melt blown polypropylene fabric; FFP2; FFP3; KF80 and/or N95 approved classes of “anti-yellow sand” masks; KF94, KF80, and/or N95 approved classes of “quarantine” masks); (II) provide an overview of the various experimental protocols in the existing literature; and (III) evaluate limitations in the current literature on mask FE. While this narrative review focuses specifically on FE, other mask performance criteria, such as breathability, reuse potential, and fit/leakage are also important considerations in face mask selection, although they are beyond the scope of this review. We present the following article in accordance with the Narrative Review reporting checklist (available at https://jphe.amegroups.com/article/view/10.21037/jphe-22-33/rc).
Methods
Relevant peer-reviewed, published literature was identified by querying the PubMed database. A summary of the search strategy is provided in Table 1. The titles of the 3,256 relevant results were screened, from which 246 titles were selected for abstract review and then 93 abstracts were selected for full text review. The references were also screened for relevant literature in the full paper review process.
Table 1
Items | Specification |
---|---|
Date of search | 8-Oct-2021 |
Databases and other sources searched | PubMed |
Search terms used | “face mask” OR “face covering” OR “cloth mask” OR “fabric mask” OR “surgical mask” OR “medical mask” OR respirator OR “KN95” OR “N95”) AND (filter OR filtration OR efficacy OR efficiency OR effectiveness) AND (aerosol OR particle OR droplet OR virus) |
Timeframe | All publications before October 8th, 2021 |
Inclusion and exclusion criteria | Inclusion: |
1. Published in peer-reviewed scientific journal† | |
2. Experimental studies of FE (i.e., PFE, BFE, VFE) of masks fabricated using readily available materials within a common household compared to a surgical mask and/or FFR (e.g., N95, KN95‡) | |
3. Described experimental methods for testing FE | |
4. Studies for all time periods and settings | |
5. Full-text article available in English language | |
Exclusion: | |
1. Studies that did not include a control surgical/procedure mask and/or FFR | |
2. Studies that reported results as particle count, pictures of aerosols, a protection factor, or other metrics that were not specifically FE as a % | |
3. Masks comprised of materials that are not readily available within a common household | |
4. Data from samples that were altered to account for mask leakage | |
5. Studies that are not representative of normal use scenarios of face masks | |
6. Studies of mask efficiency in the context of ambient air pollution | |
7. Data with reporting limitations, such as lack of information on test mask composition or measurements of FE presented graphically with no data labels | |
Selection process | Studies were screened for relevancy by two independent reviewers, first by title, and any discrepancies between the two reviewers were discussed and compared to inclusion/exclusion criteria. From there, studies were further screened by an abstract review by the same two independent reviewers. Finally, a full-paper review was conducted by three reviewers, with two people each reviewing each paper |
†, one result returned for an MMWR (Morbidity and Mortality Weekly Report) publication released by the CDC (27). According to the CDC, “[a]lthough most articles that appear in MMWR are not “peer-reviewed” in the way that submissions to medical journals are, to ensure that the content of MMWR comports with CDC policy, every submission to MMWR undergoes a rigorous multilevel clearance process before publication” (CDC, 2011). Based on our criteria for peer-reviewed, published journal articles, we did not consider any MMWR or similar government publications. ‡, KN95s are manufactured to meet the China GB2626-2006 standard, requiring a filter performance of >95% of NaCl particles, and pass a fit test (32). 3M mask manufacturer has stated that it is “reasonable” to consider China KN95 as “similar” to US NIOSH N95 masks for bioaerosols (2). The CDC, through NIOSH, does not approve KN95 masks or any other respiratory protective device certified to international standards (33). FE, filtration efficiency; PFE, particle FE; BFE, bacterial FE; VFE, viral FE; FFR, filtering facepiece respirator.
More specifically, specific data points within studies were excluded for masks comprised of materials not readily available within a common household or masks not feasible to be made in a common household, such as medical grade/surgical/sterilization wraps used in clinical settings (12,29,34), commercially manufactured/commercial grade filter materials (35,36), novel materials such as those engineered with antibacterial/antiviral properties or enhanced with triboelectric charging (1,22,37), and masks with valves, since the use of these is discouraged by the CDC (18,38-40). As stated above, we excluded samples that were altered to account for mask leakage (e.g., partially or fully taping masks, intentionally creating or simulating gaps, placing nylon overlayers and rubber bands over masks, tying ear loops and tucking in the side pleats of masks), as this review solely focused on FE (25,40-44). Data that were not representative of normal use scenarios for face masks (mechanically ventilated masks, snorkel masks, gas masks), as well as those evaluating mask efficiency in the context of particulate matter (PM10) pollution in ambient air, were not considered in this narrative review (45-47). Furthermore, we excluded data with reporting limitations such as lack of information on test mask composition (48-50); where FE was unclear for an individual mask type or material (24,49,50); and measurements of FE not being explicitly provided, but rather presented graphically with no data labels along with no, or insufficient, in-text clarification (10,19,38,41,51-54).
Results and discussion
Of the 3,256 titles reviewed, 246 were selected for abstract review, from which 96 were selected for full paper review, ultimately resulting in 31 papers that met the inclusion criteria for this narrative review. These studies include 16 US studies (12,18,22,34,36,42-44,54-61) and 15 non-US studies (i.e., Australia, Canada, China, Italy, Japan, Nepal, the Netherlands, Slovenia, South Korea, the UK) (10,11,20,29,35,40,45,47,48,62-67). Average FEs for FFRs, surgical/procedure masks, and makeshift material masks are illustrated in Figures 1-9. Average FEs were determined using the average of reported single measurements or a range of reported average measurements in the included studies. The supplemental tables present further details on particle size, flow rate, face velocity, and data reporting limitations associated with FEs for each mask type (Tables S1-S3).
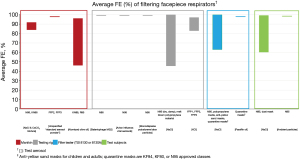
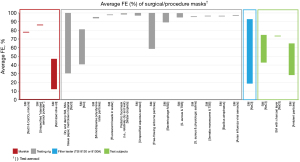
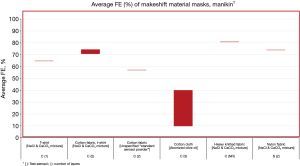
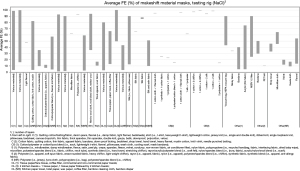
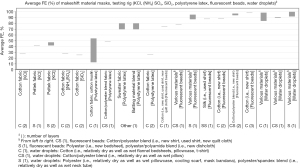
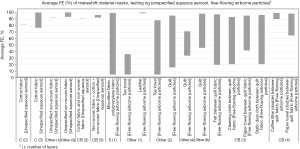
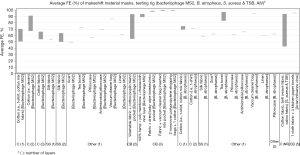
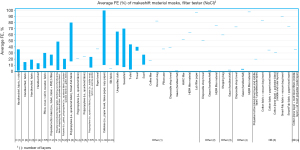
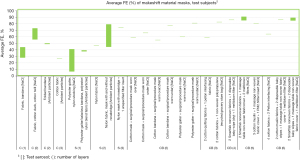
The results are stratified by studies conducted using a manikin head form, experimental testing rig, filter tester (i.e., TSI 8130 and/or TSI 8130A), and human test subjects to investigate potential trends in FE due to experimental setup (14,15). Results from each experimental category are further categorized by aerosol type. Of the included 31 studies, presented in Figures 1-9, 26 studies were conducted with particles ≤5 µm, which are defined by the WHO and CDC as aerosols or droplet nuclei (68,69). The remaining five studies (20,39,42,58,65) included test particles >5 µm, up to 20 µm, which are defined by the WHO and CDC as droplets (68,69). It is important to note that, while the WHO and CDC’s standard definitions for aerosols and droplets have been widely used, the COVID-19 pandemic has brought to light inconsistencies and inaccuracies between conventional definitions used by public health agencies and those rooted in aerosol science which more accurately characterizes different transmission routes (70,71). The principal mode by which people are infected with SARS-CoV-2 is through exposure to infectious virus-containing respiratory fluids, including through inhalation of very fine respiratory droplets and aerosol particles (72). Droplets <5 µm are generally considered the primary source of transmission in a respiratory infection, and droplets that are <1 µm may remain suspended in the air for minutes to hours (42,72). However, we did not categorize our results by aerosol size, as aerosol size was not always specified. Furthermore, several studies measured mask performance as a measure of penetration, from which we calculated FE based on Eq. [3] (24,34,48,55).
Overall, significant variability in FE was observed across all study types and mask categories. Average FE of FFRs ranged from 46% to 98% for manikin experiments, 46% to 100% for experimental testing rigs, 63% to 100% (99.96%) for filter testers, and 60% to 99% for test subject experiments (Table S1). The ranges suggest that the FE of some FFRs are notably lower than the 95% ideal efficiency of a comparative N95 respirator. There are also wide differences in filtration efficiencies as not all FFRs are subject to the same level of rigor in testing; more specifically, the performance of N95s exceeds that of non-N95 FFRs, such as KN95s, given that N95 respirators undergo NIOSH certification testing which is considered a stringent, worst-case test method to produce maximum penetration or conservative FE (21). However, it is difficult to compare FEs due to the variation in experimental setup (i.e., manikin, testing rig, filter tester, test subjects), test particles (i.e., NaCl and CaCO3 mixture, unspecified aerosol powder, atomized olive oil, bacteriophage MS2, avian influenza viral aerosols, PSL particles, NaCl, KCl, paraffin oil, unspecified ambient particles), particle size (0.012–10 µm), flow rate (0.1–95 L/min), and face velocity (4.9–1,650 cm/s). It is important to note that a high velocity such as 1,650 cm/s, simulating an adult human coughing, was achieved with a testing apparatus using flat media secured with a testing mount and adjustable clip; although this data is informative on the impact of velocity on FE, it is not comparable to studies using whole masks (e.g., whole masks on manikins or human subjects) (62). Even for experiments using the standard NaCl test aerosols specified for the NIOSH N95 respirator certification (10,18,22,29,34,43,48,55,57,62), there were deviations from the standard test procedures in particle size (0.02–2.8 µm), flow rate (0.1–95 L/min), and face velocity (4.9–1,650 cm/s). Some studies also did not report flow rate or face velocity, presenting another challenge in comparing FEs (Table S1).
The average FE of surgical/procedure masks ranged from 12% to 86% for manikin experiments, 31% to 100% for experimental testing rigs, 19% to 93% for filter testers, and 29% to 75% for test subject experiments (Table S2). While it was not always clear as to whether the surgical/procedure masks were ASTM certified, it is apparent that some of the FEs are notably lower than the 95% to 98% ideal efficiency of ASTM Level 1, 2, or 3 masks. Similar to FFRs, a variety of experimental setups (as stated above), test particles (i.e., NaCl and CaCO3 mixture, unspecified aerosol powder, atomized olive oil, NaCl, KCl, PSL particles, fluorescent beads in water, water droplets, unspecified aqueous aerosols, unspecified free-flowing airborne particles, bacteriophage MS2, Staphylococcus aureus with Trypticase Soy Broth (TSB) as well as physiologic saline, Serratia marcescens with TSB, Bacillus atrophaeus, avian influenza viral aerosols, unspecified ambient particles), particle sizes (0.01–20 µm), flow rates (0.1–95 L/min), and face velocities (1.95–1,710 cm/s) were used to evaluate FE of surgical/procedure masks; however, particle size, flow rate, and face velocity were not reported for every study. One study was identified that followed the ASTM F2299 particle type, particle size, flow rate, and face velocity criteria for PFE, and found that FE ranged from 94.2% to 94.9% for an ASTM Level 2 and Level 3 surgical mask, respectively (10). Additionally, one study was identified that followed the ASTM F2101 particle type (Staphylococcus aureus) and flow rate (~28 L/min) criteria for BFE, and found that FE ranged from 95.2% to 99.4% for surgical masks (63). While these two studies reported approximate target efficiencies for their respective test methods, the remaining 27 studies in the surgical/procedure mask category were conducted using various nonstandard methods and reported average FEs from 12.4% (40) up to 100% (29,35,65) (Table S2).
Average FE of makeshift materials ranged from 9.8% to 81% for manikin experiments, 1.5% to 100% for experimental testing rigs, 0.43% to 100% (99.85%) for filter testers, and 7.0% to 91% for test subject experiments (Table S3). The results were stratified by material composition (i.e., cotton or cotton-based, cotton/synthetic blends, synthetic or synthetic-based, cellulose or cellulose-based, silk/satin, fabric combinations, wool, and other makeshift materials that do not belong to any of the aforementioned categories), number of layers, test particles (i.e., NaCl and CoCO3 mixture, unspecified aerosol powder, atomized olive oil, NaCl, KCl, (NH4)2SO4, SiO2, PSL particles, fluorescent beads in water, water droplets, unspecified aqueous aerosols, unspecified free-flowing airborne particles, bacteriophage MS2, Bacillus atrophaeus, Staphylococcus aureus with TSB, avian influenza viral aerosols, unspecified ambient particles), particle size (0.01–20 µm), flow rate (0.1–99 L/min), and face velocity (0.26–1,710 cm/s); of note, some studies did not report particle size, flow rate, or face velocity (Table S3).
Overall, FE is generally the highest across all experimental categories in FFRs (45.68–100%), as expected, followed by surgical/procedure masks (12.4–100%) and then makeshift materials (0.433–100%). Interestingly, the lower bound FEs for the FFR, surgical/procedure mask, and makeshift barrier face covering categories are all notably lower than their target efficiencies (i.e., ≥95% for an N95, ≥95–98% for ASTM Level 1–3 surgical masks, and ≥20% (Level 1) or ≥50% (Level 2) for barrier face coverings). For example, the lowest reported FE for FFRs was an average of 45.68%, which was measured when a damp N95 was challenged with 0.02 to 0.1 µm NaCl under high velocity conditions of 1,650 cm/s to simulate an adult coughing (flow rate not reported) (62). For surgical masks, the lowest reported average FE of 12.4% was measured when a surgical mask was challenged with 1 µm atomized olive oil at a flow rate of 24 L/min and face velocity of 10 to 12 cm/s (40). For makeshift materials, the lowest average FE of 0.433% was measured when a single layer gauze handkerchief was challenged with 0.0779 µm NaCl at a flow rate of 95 L/min and unreported face velocity (48).
Conversely, an FE of 100% was achieved in all three respirator/mask categories. The highest reported FE for FFRs of 100% represents average FE of 2.8 µm NaCl for an N95 at a flow rate of 1 L/min and face velocity of 4.9 cm/s (29). An FE of 100% was achieved for surgical masks and surgical mask/makeshift material combinations using various test particles (i.e., NaCl, unspecified aqueous aerosols, free-flowing airborne particles), particle sizes (i.e., 2–>5 µm), flow rates (1–28.2 L/min), and face velocities (4.9 cm/s; NR for two of three studies) (29,35,65). For makeshift materials, 100% FE was achieved for a 3-layer cotton fabric, 3-layer unspecified non-woven fabric, and 1-layer leather using various test particles (unspecified aqueous aerosols and free-flowing airborne particles), particle sizes (0.3–>5 µm), and flow rates (6–28.32 L/min); face velocities were not reported for any of these samples (35,65).
A wide range in FE is observed for all mask categories, some ranging well below the required efficiencies specified by NIOSH and ASTM standards for the respective respirator/mask types all the way up to 100%. The results demonstrate that FE is a complex interplay between material composition, material construction (yarn count, fabric mass, weave type), challenge aerosol characteristics (particle size, composition), and velocity of the air passing through the filter (12,73,74). Therefore, it is difficult to compare results due to the wide variability in test methodologies as well as the lack of reporting of various study parameters in some studies such as particle size, flow rate, and face velocity.
Notably, some tests were conducted using oil-based aerosols (olive oil, paraffin oil). Electret filters, such as N95s, rely heavily on electrostatic charge to achieve target filtration efficiencies. It has been demonstrated that in the presence of oil-based aerosols, electret filter fibers become coated in oil, resulting in the degradation of filter media and increased filter penetration (75). Only certain filter media have been specifically designed to be resistant to oil (e.g., NIOSH R-series) or strongly resistant to oil (e.g., NIOSH P-series) (23). As such, the results of tests conducted using oil-based aerosols on filtration media not specifically designed for oil resistance are not a reliable indicator of filtration media performance in the presence of non-oil particulates.
Face velocity and filtration mechanisms
Higher face velocities have been shown to reduce FE; however, the impact of this variable on FE also depends on the filtration mechanism (42,62,74,76). Although there is a perception that fibrous filters behave like a sieve in which particles above a certain size are trapped while smaller particles pass through, data have shown a more complex interplay between FE and particle size (73). Due to the physical mechanisms of diffusion, interception, and inertial impaction, fibrous filters trap smaller and larger particles more effectively, whereas the most penetrating particle size (MPPS) falls within the intermediate range (12,39,73,74). Diffusion is the main filtration mechanism for smaller particles (<0.1 µm) characterized by random, zigzagging motions (Brownian motion). Therefore, the smaller the particle and the lower the velocity, the higher the chances of the randomly moving particles to contact and stick to a filter fiber (73). For larger particles ≥0.4 µm, filters are efficient due to the predominance of interception and inertial impaction (73). With interception, when a particle following a gas streamline comes within one particle radius of a filter fiber, the particle is captured by the fiber upon contact. With inertial impaction, the sheer size of a particle prohibits it from adjusting to changes in a gas streamline near a filter fiber, allowing the filter fiber to capture the particle as it continues moving along its original path (73). The MPPS is approximately 0.05 to 0.5 µm, in which particles are too small for interception and too large for diffusion, thereby resulting in a U-shaped curve when FE is plotted against (12,39,73,74). For comparison purposes, studies have shown the SARS-CoV-2 virus within this intermediate region of approximately 0.06 to 0.14 µm in diameter; notably, the SARS-CoV-2 virus will be contained within droplets of various sizes (77-79). Of note, while it is important to distinguish between physical diameter and aerodynamic diameter (i.e., diameter of a sphere of unit density with the same settling velocity as the particle) in order to characterize particle behavior, the studies reviewed did not provide such level of detail.
Material composition, construction, and layers
It is especially challenging to compare the FE of makeshift materials due to the additional variability in material composition, construction (tightness of weave, fiber intervals, fabric structure), and number of layers. In general, cloth masks with a higher weave density have higher FE (42,54,58). For example, an increase was observed from 9-14% FE for 80 threads per inch (TPI) cotton to 79–98.4% FE for 600 TPI cotton and from 8.7% FE for 600 TPI cotton to 48.95–53.34% FE for 1,000 TPI cotton (42,58). Multi-layered masks also generally performed better than single-layer masks (12,22,42,56,62). Although high FEs can be achieved based on some of these material qualities, some materials are not practical due to poor fit/leakage and high pressure drop. For example, 99–100% FE was achieved with leather and 99.8% for copy paper, although neither materials are good candidates for homemade masks due to high pressure drops, an indicator of poor breathability (22,35). While more layers are generally associated with higher FE, breathability becomes an issue as the differential pressure increases (12). When the differential pressure increases, the higher drop in pressure increases face seal leaks and reduces mask performance because the increase in flow resistance forces more air flow out of the leaks versus the mask material as intended. Another important consideration with cloth materials is the effect of washing on FE. Given the reusable nature of cloth masks, they should be washed at least once per day or as soon as they become wet or dirty (80). However, there is evidence that the pores of cotton fabric widen after washing, altering the material quality and filtration ability (35).
Mixed makeshift material assemblies
Some studies reported increased FE for masks comprised of layers made of different makeshift materials. For example, Konda et al. reported >80% to >90% FE for cotton-silk, cotton-chiffon, and cotton-flannel hybrids (42). Amendola et al. reported an increase in FE from 79–83% for a two-layered cotton mask to 90–92% for a two-layered cotton/non-woven fabric mask, as well as 77–83% for a three-layered cotton mask to 92–96% for a three layered non-woven/cotton/non-woven mask (65). Further, O’Kelly reported an increase in FE from 10–47% for single-layer masks to 35–60% for masks with more than one type of fabric or heat-bonded with a fusible interfacing (62). Although Zangmeister et al. did not identify a significant difference in mixed cloth assemblies compared to individual components, enhanced performance, when observed, of hybrid material masks may be attributed to the combined mechanical and electrostatic-based filtration effects (12,42).
Effect of humidity/dampness and potential hazards of makeshift materials
Another factor that affects filtration performance is dampness, such as from exercise, respiration, and speech. O’Kelly et al. reported only minor differences in FE for quilting cotton, cotton flannel, and craft felt while damp (dry: 28.5–34.54%, damp: 30.14–31.88%); however, a significant decrease in FE was observed in damp denim (dry: 45.95%, damp: 30.68%) while an increase was observed for high efficiency particulate air (HEPA) single-use vacuum bags (dry: 60.86%, damp: 71.93%) (62). However, Guha et al. reported no significant changes in wet efficiency over time (dry → partially wet → wet), implying that fabric materials may continuously offer protection over the course of multiple sneezes or coughs, and humidity from exhaled breath may not significantly impact FE (58). On the contrary, Zangmeister et al. reported an increase in FE of hydrophilic fabrics when challenged with hygroscopic nanoparticles, such as respiratory droplets containing SARS-CoV-2, under high humidity conditions (59).
Certain makeshift mask materials may pose potential hazards to individuals making or wearing such masks. For instance, O’Kelly et al. reported that while the single-use HEPA vacuum bag showed the high FE of ultrafine particles, the layers fell apart when the material was cut, exposing component materials that may be unsafe for individuals to inhale or come into close contact with the face. The authors noted that manipulating a reusable, washable HEPA bag did not expose the inner fibers, but any potential hazards of such materials are unknown (62). Flame retardant properties, skin irritation, and delayed-type hypersensitivity of mask materials are also important areas for further investigation (81).
Conclusions
As the COVID-19 pandemic enters its second year, researchers continue to help advance health and safety measures to prevent the transmission of the SARS-CoV-2 virus. One such measure, improvised face masks, has been studied to understand FE for various materials available to the general population, although the findings are highly variable and difficult to compare. Overall, no single filter characteristic is a sole determinant of FE, and a combination of factors, such as those described above (e.g., face velocity, particle size and type, material composition, material construction, layers) contribute to differences in filter quality (54). Based on the available data, the FE of surgical/procedure masks is generally higher than cloth masks, and FFRs are the highest of all, as expected. However, it is challenging to make direct comparisons across these studies due to the variations in testing methods, many of which lack standardization, as well as study reporting limitations. The wide variability in FE in makeshift materials suggests that material properties that contribute to high FEs are still poorly understood (10). However, it is generally understood that wearing improvised masks is better for viral emission reduction than being totally unprotected, and various techniques (e.g., increasing layers, using higher weave density materials, improving fit to reduce leakage) can help increase FE of improvised masks (24,36,42,54,58).
Another uncertainty when comparing the results of these studies is the variability in the reported data (e.g., as characterized by the standard deviation). Not all studies reported a standard deviation (34,65), some studies had a small range [0.00–0.066 (56); 0.68–2.65 (64)], and other studies had a large range [3–16 (11); 0.5–13.9 (44)]. A large standard deviation indicates data points far from the mean, and may be demonstrative of a wide variation in the FE of the material samples. Large variations in FE are particularly apparent for makeshift materials, for which filtration characteristics are not controlled. Varying standard deviations indicate a lack of accuracy in the tests, which further speaks to the need for more comprehensive data reporting and standardization in test methods. Future research efforts can aim to use more standardized experimental methods (e.g., NIOSH NaCl method for N95 certification, ASTM F3502-21) to reduce variability in study protocols, improve on data reporting by providing quantifiable results (as opposed to presenting in graphs with no data labels or table/in-text clarification), and clarifying study parameters (e.g., flow rate, face velocity, particle type and size, mask layers and composition). In particular with homemade face masks, the new ASTM F3502-21 Barrier Face Covering Standard was developed to establish uniform test methods and performance criteria to allow for more effective comparison across a wide variety of makeshift mask materials; the standard also provides design requirements for the general construction of masks, use of nonirritating and nontoxic materials, flammability, sizing, and shelf life. These measures will help improve readability and like-for-like comparisons across different studies.
Acknowledgments
The authors would like to thank Carrie Kahn (Cardno ChemRisk) for library science support.
Funding: None.
Footnote
Provenance and Peer Review: This article was commissioned by the Guest Editors (Kenneth A. Mundt and Justine Parker) for the series “Managing COVID-19 Risks in the Workplace” published in Journal of Public Health and Emergency. The article has undergone external peer review.
Reporting Checklist: The authors have completed the Narrative Review reporting checklist. Available at https://jphe.amegroups.com/article/view/10.21037/jphe-22-33/rc
Conflicts of Interest: All authors have completed the ICMJE uniform disclosure form (available at https://jphe.amegroups.com/article/view/10.21037/jphe-22-33/coif). The series “Managing COVID-19 Risks in the Workplace” was commissioned by the editorial office without any funding or sponsorship. All authors are currently employed by Cardno ChemRisk. The authors have no other conflicts of interest to declare.
Ethical Statement: The authors are accountable for all aspects of the work in ensuring that questions related to the accuracy or integrity of any part of the work are appropriately investigated and resolved.
Open Access Statement: This is an Open Access article distributed in accordance with the Creative Commons Attribution-NonCommercial-NoDerivs 4.0 International License (CC BY-NC-ND 4.0), which permits the non-commercial replication and distribution of the article with the strict proviso that no changes or edits are made and the original work is properly cited (including links to both the formal publication through the relevant DOI and the license). See: https://creativecommons.org/licenses/by-nc-nd/4.0/.
References
- Siller P, Reissner J, Hansen S, et al. Innovative Textiles Used in Face Masks: Filtration Efficiency and Self-Disinfecting Properties against Coronaviruses. Nanomaterials (Basel) 2021;11:2088. [Crossref] [PubMed]
- WHO. WHO Director-General's Opening Remarks at the Media Briefing on COVID-19 - 11 March 2020. Retrieved Jan. 23, 2022. Available online: https://www.who.int/director-general/speeches/detail/who-director-general-s-opening-remarks-at-the-media-briefing-on-covid-19---11-march-2020
- Face Masks FDA. Barrier Face Coverings, Surgical Masks, and Respirators for COVID-19. Content Current as of Sep. 2021;15:
- 3M. The Differences Between Masks and Respirators. Sep. 25, 2020. Updated Oct. 5, 2020. Retrieved April 7, 2022. Available online: https://news.3m.com/The-differences-between-masks-and-respirators
- World Health Organization. (2020). Advice on the use of masks in the context of COVID-19: interim guidance, 6 April 2020. Retrieved April 27, 2022. Available online: https://apps.who.int/iris/handle/10665/331693
- CDC. COVID-19: Types of Masks and Respirators. Updated Jan. 28, 2022. Retrieved April 7, 2022. Available online: https://www.cdc.gov/coronavirus/2019-ncov/prevent-getting-sick/types-of-masks.html
- FDA. N95 Respirators, Surgical Masks, Face Masks, and Barrier Face Coverings. Content Current as of Sept. 15, 2021. Retrieved Jan. 23, 2022. Available online: https://www.fda.gov/medical-devices/personal-protective-equipment-infection-control/n95-respirators-surgical-masks-face-masks-and-barrier-face-coverings
- ASTM. ASTM F3502-21: Standard Specification for Barrier Face Coverings. Last Updated Feb. 15, 2021. Retrieved Jan. 23, 2022. Available online: https://www.astm.org/f3502-21.html
- Szalajda J, Stull JO, Brosseau LM. NIOSH Science Blog: Overview of the ASTM F3502-21 Barrier Face Covering Standard. April 23, 2021. Page Last Updated Aug. 11, 2021. Retrieved Jan. 23, 2022. Available online: https://blogs.cdc.gov/niosh-science-blog/2021/04/23/bfc-standard/
- LaRue RJ, Morkus P, Laengert S, et al. Navigating Performance Standards for Face Mask Materials: A Custom-Built Apparatus for Measuring Particle Filtration Efficiency. Glob Chall 2021;5:2100052. [Crossref] [PubMed]
- Sharma A, Omidvarborna H, Kumar P. Efficacy of facemasks in mitigating respiratory exposure to submicron aerosols. J Hazard Mater 2022;422:126783. [Crossref] [PubMed]
- Zangmeister CD, Radney JG, Vicenzi EP, et al. Filtration Efficiencies of Nanoscale Aerosol by Cloth Mask Materials Used to Slow the Spread of SARS-CoV-2. ACS Nano 2020;14:9188-200. [Crossref] [PubMed]
- Forouzandeh P, O'Dowd K, Pillai SC. Face masks and respirators in the fight against the COVID-19 pandemic: An overview of the standards and testing methods. Saf Sci 2021;133:104995. [Crossref] [PubMed]
- NIOSH. Determination of Particulate Filter Efficiency Level for N95 Series Filters against Solid Particulates for Non-Powered, Air-Purifying Respirators Standard Testing Procedure (STP) (TEB-APR-STP-0059). Washington DC: National Institute for Occupational Safety and Health; 2019.
- CDC. How to Tell if your N95 Respirator is NIOSH Approved. NIOSH Pub. No.: 2021-124. Sep. 2021. Retrieved April 27, 2022. Available online: https://www.cdc.gov/niosh/docs/2021-124/default.html?msclkid=6d66daf7c68511ecb8cc5d1e864539d0
- Nelson Labs. Bacterial & Viral Filtration Efficiency (BFE/VFE). Retrieved Jan. 24, 2022. Available online: https://www.nelsonlabs.com/testing/bacterial-viral-filtration-efficiency-bfe-vfe/
- Corbin JC, Smallwood GJ, Leroux ID, et al. Systematic experimental comparison of particle filtration efficiency test methods for commercial respirators and face masks. Sci Rep 2021;11:21979. [Crossref] [PubMed]
- Lindsley WG, Blachere FM, Beezhold DH, et al. A comparison of performance metrics for cloth masks as source control devices for simulated cough and exhalation aerosols. Aerosol Sci Technol 2021;55:1125-42. [Crossref] [PubMed]
- Sheets D, Shaw J, Baldwin M, et al. An apparatus for rapid and nondestructive comparison of masks and respirators. Rev Sci Instrum 2020;91:114101. [Crossref] [PubMed]
- Whiley H, Keerthirathne TP, Nisar MA, et al. Viral Filtration Efficiency of Fabric Masks Compared with Surgical and N95 Masks. Pathogens 2020;9:762. [Crossref] [PubMed]
- Rengasamy S, Shaffer R, Williams B, et al. A comparison of facemask and respirator filtration test methods. J Occup Environ Hyg 2017;14:92-103. [Crossref] [PubMed]
- Zhao M, Liao L, Xiao W, et al. Household Materials Selection for Homemade Cloth Face Coverings and Their Filtration Efficiency Enhancement with Triboelectric Charging. Nano Lett 2020;20:5544-52. [Crossref] [PubMed]
- CDC. Respirator Fact Sheet. Page Last Reviewed April 9, 2020. Retrieved Jan. 24, 2022. Available online: https://www.cdc.gov/niosh/npptl/topics/respirators/factsheets/respsars.html
- Duncan S, Bodurtha P, Naqvi S. The protective performance of reusable cloth face masks, disposable procedure masks, KN95 masks and N95 respirators: Filtration and total inward leakage. PLoS One 2021;16:e0258191. [Crossref] [PubMed]
- Cooper DW, Hinds WC, Price JM, et al. Common materials for emergency respiratory protection: leakage tests with a manikin. Am Ind Hyg Assoc J 1983;44:720-6. [Crossref] [PubMed]
- CDC. Health and Safety Practices Survey of Healthcare Workers: Use of Respirators and Surgical Masks for Protection Against Healthcare Hazards. Page Last Reviewed Nov. 19, 2018. Retrieved Jan. 23, 2022. Available online: https://www.cdc.gov/niosh/topics/healthcarehsps/respiratory.html#:~:text=Respirators
- Brooks JT, Beezhold DH, Noti JD, et al. Maximizing Fit for Cloth and Medical Procedure Masks to Improve Performance and Reduce SARS-CoV-2 Transmission and Exposure, 2021. MMWR Morb Mortal Wkly Rep 2021;70:254-7. [Crossref] [PubMed]
- Bhattacharjee S, Bahl P, Chughtai AA, et al. Last-resort strategies during mask shortages: optimal design features of cloth masks and decontamination of disposable masks during the COVID-19 pandemic. BMJ Open Respir Res 2020;7:e000698. [Crossref] [PubMed]
- Rogak SN, Sipkens TA, Guan M, et al. Properties of materials considered for improvised masks. Aerosol Sci Tech 2021;55:398-413. [Crossref]
- Krishan B, Gupta D, Vadlamudi G, et al. Efficacy of homemade face masks against human coughs: Insights on penetration, atomization, and aerosolization of cough droplets. Phys Fluids (1994) 2021;33:093309.
- Godoy M. The Coronavirus Crisis: With Omicron, You Need A Mask That Means Business. Dec. 23, 2021. Retrieved Jan. 23, 2022. Available online: https://www.npr.org/sections/health-shots/2021/12/23/1066871176/mask-n95-omicron-contagious
- 3M. Technical Bulletin: Comparison of FFP2, KN95, and N95 Filtering Facepiece Respirator Classes. Rev. 6. Feb., 2021. St. Paul, MN: 3M Co.; 2021.
- CDC. Counterfeit Respirators/Misrepresentation of NIOSH-Approval. Page Last Reviewed March 17, 2022. Retrieved March 24, 2022. Available online: https://www.cdc.gov/niosh/npptl/usernotices/counterfeitResp.html
- Jones IF, Lammers DT, Conner JR, et al. Quality Assurance During a Global Pandemic: An Evaluation of Improvised Filter Materials for Healthcare Workers. J Occup Environ Med 2020;62:781-2. [Crossref] [PubMed]
- Teesing GR, van Straten B, de Man P, et al. Is there an adequate alternative to commercially manufactured face masks? A comparison of various materials and forms. J Hosp Infect 2020;106:246-53. [Crossref] [PubMed]
- Reutman SR, Reponen T, Yermakov M, et al. Homemade facemasks: particle filtration, breathability, fit, and other performance characteristics. J Occup Environ Hyg 2021;18:334-44. [Crossref] [PubMed]
- Zhang Z, Ji D, He H, et al. Electrospun ultrafine fibers for advanced face masks. Mater Sci Eng R Rep 2021;143:100594. [Crossref] [PubMed]
- Shakya KM, Noyes A, Kallin R, et al. Evaluating the efficacy of cloth facemasks in reducing particulate matter exposure. J Expo Sci Environ Epidemiol 2017;27:352-7. [Crossref] [PubMed]
- Patra SS, Nath J, Panda S, et al. Evaluating the filtration efficiency of commercial facemasks' materials against respiratory aerosol droplets. J Air Waste Manag Assoc 2022;72:3-9. [Crossref] [PubMed]
- Shah Y, Kurelek JW, Peterson SD, et al. Experimental investigation of indoor aerosol dispersion and accumulation in the context of COVID-19: Effects of masks and ventilation. Phys Fluids (1994) 2021;33:073315.
- Hill WC, Hull MS, MacCuspie RI. Testing of Commercial Masks and Respirators and Cotton Mask Insert Materials using SARS-CoV-2 Virion-Sized Particulates: Comparison of Ideal Aerosol Filtration Efficiency versus Fitted Filtration Efficiency. Nano Lett 2020;20:7642-7. [Crossref] [PubMed]
- Konda A, Prakash A, Moss GA, et al. Aerosol Filtration Efficiency of Common Fabrics Used in Respiratory Cloth Masks. ACS Nano 2020;14:6339-47. [Crossref] [PubMed]
- Mueller AV, Eden MJ, Oakes JM, et al. Quantitative Method for Comparative Assessment of Particle Removal Efficiency of Fabric Masks as Alternatives to Standard Surgical Masks for PPE. Matter 2020;3:950-62. [Crossref] [PubMed]
- Clapp PW, Sickbert-Bennett EE, Samet JM, et al. Evaluation of Cloth Masks and Modified Procedure Masks as Personal Protective Equipment for the Public During the COVID-19 Pandemic. JAMA Intern Med 2021;181:463-9. [Crossref] [PubMed]
- Neupane BB, Mainali S, Sharma A, et al. Optical microscopic study of surface morphology and filtering efficiency of face masks. PeerJ 2019;7:e7142. [Crossref] [PubMed]
- Huo S, Zhang TT. Ventilation of ordinary face masks. Build Environ 2021;205:108261. [Crossref] [PubMed]
- Pogačnik Krajnc A, Pirker L, Gradišar Centa U, et al. Size- and Time-Dependent Particle Removal Efficiency of Face Masks and Improvised Respiratory Protection Equipment Used during the COVID-19 Pandemic. Sensors (Basel) 2021;21:1567. [Crossref] [PubMed]
- Jung H, Kim J, Lee S, et al. Comparison of filtration efficiency and pressure drop in anti-yellow sand masks, quarantine masks, medical masks, general masks, and handkerchiefs. Aerosol Air Qual Res 2014;14:991-1002. [Crossref]
- Lee EH, Lee SW, Moon SY, et al. Performance Evaluation of Commercially Available Masks in Korea for Filtering Airborne Droplets Containing Bacteria. Int J Environ Res Public Health 2021;18:7909. [Crossref] [PubMed]
- Sterr CM, Nickel IL, Stranzinger C, et al. Medical face masks offer self-protection against aerosols: An evaluation using a practical in vitro approach on a dummy head. PLoS One 2021;16:e0248099. [Crossref] [PubMed]
- Liu Z, Yu D, Ge Y, et al. Understanding the factors involved in determining the bioburdens of surgical masks. Ann Transl Med 2019;7:754. [Crossref] [PubMed]
- Hao W, Parasch A, Williams S, et al. Filtration performances of non-medical materials as candidates for manufacturing facemasks and respirators. Int J Hyg Environ Health 2020;229:113582. [Crossref] [PubMed]
- Leite KFS, Cheng K, Kumar S, et al. Máscarasdo Bem: An analysis of face-mask performance from a volunteer mask-making initiative in Ribeirão Preto, Brazil. Public Health Pract (Oxf) 2021;2:100094. [Crossref] [PubMed]
- Pan J, Harb C, Leng W, et al. Inward and outward effectiveness of cloth masks, a surgical mask, and a face shield. Aerosol Sci Tech 2021;55:718-33. [Crossref]
- Rengasamy S, Eimer B, Shaffer RE. Simple respiratory protection--evaluation of the filtration performance of cloth masks and common fabric materials against 20-1000 nm size particles. Ann Occup Hyg 2010;54:789-98. [PubMed]
- Aydin O, Emon B, Cheng S, et al. Performance of fabrics for home-made masks against the spread of COVID-19 through droplets: A quantitative mechanistic study. Extreme Mech Lett 2020;40:100924. [Crossref] [PubMed]
- Long KD, Woodburn EV, Berg IC, et al. Measurement of filtration efficiencies of healthcare and consumer materials using modified respirator fit tester setup. PLoS One 2020;15:e0240499. [Crossref] [PubMed]
- Guha S, Herman A, Carr IA, et al. Comprehensive characterization of protective face coverings made from household fabrics. PLoS One 2021;16:e0244626. [Crossref] [PubMed]
- Zangmeister CD, Radney JG, Staymates ME, et al. Hydration of Hydrophilic Cloth Face Masks Enhances the Filtration of Nanoparticles. ACS Appl Nano Mater 2021;4:2694-701. [Crossref] [PubMed]
- Hao W, Xu G, Wang Y. Factors influencing the filtration performance of homemade face masks. J Occup Environ Hyg 2021;18:128-38. [Crossref] [PubMed]
- Sickbert-Bennett EE, Samet JM, Prince SE, et al. Fitted filtration efficiency of double masking during the COVID-19 Pandemic. JAMA Intern Med 2021;181:1126-8. [Crossref] [PubMed]
- O'Kelly E, Pirog S, Ward J, et al. Ability of fabric face mask materials to filter ultrafine particles at coughing velocity. BMJ Open 2020;10:e039424. [Crossref] [PubMed]
- Furuhashi M. A study on the microbial filtration efficiency of surgical face masks--with special reference to the non-woven fabric mask. Bull Tokyo Med Dent Univ 1978;25:7-15. [PubMed]
- Davies A, Thompson KA, Giri K, et al. Testing the efficacy of homemade masks: would they protect in an influenza pandemic? Disaster Med Public Health Prep 2013;7:413-8. [Crossref] [PubMed]
- Amendola L, Saurini MT, Di Girolamo F, et al. A rapid screening method for testing the efficiency of masks in breaking down aerosols. Microchem J 2020;157:104928. [Crossref] [PubMed]
- Li IW, Fan JK, Lai AC, et al. Home-made masks with filtration efficiency for nano-aerosols for community mitigation of COVID-19 pandemic. Public Health 2020;188:42-50. [Crossref] [PubMed]
- Ma QX, Shan H, Zhang HL, et al. Potential utilities of mask-wearing and instant hand hygiene for fighting SARS-CoV-2. J Med Virol 2020;92:1567-71. [Crossref] [PubMed]
- Siegel JD, Rhinehart E, Jackson M, et al. 2007 Guideline for Isolation Precautions: Preventing Transmission of Infectious agents in Healthcare Settings (2007). Last Update July, 2019. Retrieved April 27, 2022. Available online: https://www.cdc.gov/infectioncontrol/guidelines/isolation/index.html
- WHO. Modes of Transmission of Virus Causing COVID-19: Implications for IPC Precautions Recommendations: Scientific Brief. March 29, 2020. Retrieved April 22, 2022. Available online: https://www.who.int/news-room/commentaries/detail/modes-of-transmission-of-virus-causing-covid-19-implications-for-ipc-precaution-recommendations
- Marr LC, Tang JW. A Paradigm Shift to Align Transmission Routes With Mechanisms. Clin Infect Dis 2021;73:1747-9. [Crossref] [PubMed]
- Tang JW, Bahnfleth WP, Bluyssen PM, et al. Dismantling myths on the airborne transmission of severe acute respiratory syndrome coronavirus-2 (SARS-CoV-2). J Hosp Infect 2021;110:89-96. [Crossref] [PubMed]
- CDC. Scientific Brief: SARS-CoV-2 Transmission. Updated May 7, 2021. Retrieved April 4, 2022. Available online: https://www.cdc.gov/coronavirus/2019-ncov/science/science-briefs/sars-cov-2-transmission.html
- TSI Inc. Mechanisms of Filtration for High Frequency Efficiency Fibrous Filters. Application Note ITI-041 (A4). Retrieved April 27, 2022. Available online: https://tsi.com/getmedia/4982cf03-ea99-4d0f-a660-42b24aedba14/ITI-041-A4?ext=.pdf
- Dhanraj DIA, Choudhary S, Jammalamadaka U, et al. Size-Dependent Filtration Efficiency of Alternative Facemask Filter Materials. Materials (Basel) 2021;14:1868. [Crossref] [PubMed]
- Martin SB Jr, Moyer ES. Electrostatic respirator filter media: filter efficiency and most penetrating particle size effects. Appl Occup Environ Hyg 2000;15:609-17. [Crossref] [PubMed]
- Whyte HE, Montigaud Y, Audoux E, et al. Comparison of bacterial filtration efficiency vs. particle filtration efficiency to assess the performance of non-medical face masks. Sci Rep 2022;12:1188. [Crossref] [PubMed]
- Kim JM, Chung YS, Jo HJ, et al. Identification of Coronavirus Isolated from a Patient in Korea with COVID-19. Osong Public Health Res Perspect 2020;11:3-7. [Crossref] [PubMed]
- Zhu N, Zhang D, Wang W, et al. A Novel Coronavirus from Patients with Pneumonia in China, 2019. N Engl J Med 2020;382:727-33. [Crossref] [PubMed]
- Wang Y, Xu G, Huang YW. Modeling the load of SARS-CoV-2 virus in human expelled particles during coughing and speaking. PLoS One 2020;15:e0241539. [Crossref] [PubMed]
- CDC. COVID-19: Use and Care of Masks. Updated Feb. 25, 2022. Retrieved April 20, 2022. Available online: https://www.cdc.gov/coronavirus/2019-ncov/prevent-getting-sick/about-face-coverings.html
- Wang D, You Y, Zhou X, et al. Selection of homemade mask materials for preventing transmission of COVID-19: A laboratory study. PLoS One 2020;15:e0240285. [Crossref] [PubMed]
Cite this article as: Louie F, Garnick L, Hernandez AM, Gaffney SH, Zisook RE. Filtration efficiency of makeshift material masks vs. surgical/procedure masks and/or filtering facepiece respirators: a narrative review. J Public Health Emerg 2022;6:36.